There are a number of intermediate genes where the mutation apparently produces a partly active form of tyrosinase. Some C alleles known in other mammals are:
- C full color, allows full expression of whatever pigment is prescribed by other genes. Most dogs are CC.
- c, chinchilla or silver, when present in double dose removes most or all of the phaeomelanin pigment with only a slight effect on black pigment. This is named after a small fur-bearing South American rodent called the chinchilla. Black and silver replacing black and tan, or a wolf-like color without the extra banding (see aw, above) may also be due to a cchcch genotype. Dogs with very light tan probably are cchcch or something similar. Liver dogs show lightening even of eumelanin pigment, and the "deadgrass" color of the Chesapeake Bay Retriever is thought to be due to a bbcchcchgenetic makeup. The possibility of other, rufous modifiers affecting the shade of phaeomelanin pigment needs to be kept in mind, as does the possibility of more than one form of chinchilla in the dog - rabbits are thought to have three.
- ce, extreme dilution, has also been proposed for the dog. This gene may be part of the makeup of some "white" dog breeds where the white color is due to extreme dilution of tan. The West Highland White Terrier may be ceceee. A cross to a black and tan breed would be interesting from the point of view of color genetics. Eyes may be lightened in some species, but this is doubtful in dogs.
- ch, Himalyan, is not known to occur in the dog. In homozygous form, it makes the formation of eumelanin dependant on the temperature of the skin. Thus a genetically solid black animal will have reduced black on the extremities (seal brown) and an almost white color on the body. The effect on tan/orange pigment is confusing - the tan in agouti hairs is removed, but that resulting from the orange gene in cats (not in dogs) remains intense on the extremities. There is reason to suspect that this gene, as well as some forms of chinchilla, also affects the organization of the brain, particularly in the neural pathways from the eyes to the brain. There may be a reason for Siamese cats to be cross-eyed. Eyes are normally blue or pink.
- cp, platinum, is optically similar to Ice White but retains very slight tysonase activity and in the mouse is described as retaining some luster in the coat as opposed to the pure white seen in Ice White .
- c, albino, is known to occur in the dog RARELY , though possible candidates for mutations to c have been recorded. As mentioned above, the c gene cannot produce working tyrosinase, and a cc individual cannot produce melanin pigment.
As seen from the above, C is known to have a number of different forms and effects. The usual assumption is that dogs have at least one mutant allele, cchwhich when homozygous lightens phaeomelanin (yellow) pigment to cream and more weakly affects liver and black. A second proposed allele, ce may be responsible for further reduction of cream to white in some breeds, or modifying alleles may be responsible for the further lightening in these cases. While some forms of C modify eye pigment. C appears to be fully dominant over any of the other alleles, the dominance relationship between the others generally goes in the direction of more color incompletely dominant over less color, the heterozygote generally resembling but not necessarily identical to the homozygote with more pigment
D, the dilution series. This, again, is a relatively simple series, containing D (dominant, full pigmentation) and d (recessive, dilute pigment). In contrast to C, which has its strongest effect on phaeomelanin, or B, which effects only eumelanin, D affects both eumelanin and phaeomelanin pigment. It is thought to act by causing the clumping of pigment granules in the hair. Like B, it often affects skin and eye color.... blue" is a term often used to describe dd blacks. If a solid liver dog also is dd, the result is the silvery color known as Lavendar .
While dd acting on black or liver is a part of the genotype of several breeds, dd acting on sable is relatively rare. For one thing, the action of dd on phaeomelanin has been described as a flattening or dulling of colorCanine Color Genetics
BY
The pigment that is responsible for a dog’s hair color is called melanin. There are two types of melanin in dogs: a pigment associated with black and brown coloration (called eumelanin) and a pigment associated with lighter colors such as yellow, red and orange (called phaeomelanin). There are a number of genes that affect how and where these pigments are produced. The genes can occur as variant forms, called alleles. Some alleles play a dominant role (usually identified by capital letters) over others and their presence will overshadow the effect of recessive alleles (identified by small letters).
The gene designated as E is responsible for eumelanin production. If the dominant form of E is not present (ee genotype), the black and brown pigment is not produced and the dog’s coat will be reddish in color (yellow, orange or red). On the other hand, if at least one E allele (EE or Ee genotypes) occurs, black or brown pigment would be the basis for a dog’s coat color. A third allele, EM, causes reddish dogs to have a black or brown facial mask (Briards, Italian Grehounds). An EG allele is necessary for Afghan Hounds to show the pattern known as “domino” and for Salukis to show the pattern known as “grizzle”. The dominance hierarchy is EM > EG > E > e at this locus.
The absence of the dominant form of B will change the black color of eumelanin into brown but will have no effect on the coat color of ee genotype dogs. For example, dogs with EEBB, EeBB, EeBb genotypes will have eumelanin normally produced (E is present) and will have black coat color (the dominant B is present). EEbb and Eebb genotypes will have eumelanin produced but their coat will be brown in color (B is not present). Note that any bb genotype dog will have brown nose and pad leather. The eeBB, eeBb, and eebb genotype dogs do not produce eumelanin pigment and will have reddish coat color.
The A gene is a bit more complicated. This gene controls where and how two pigments are produced on the body or even on the individual hair. It is very important to understand that the effects of this gene depend on the ability to produce eumelanin, which does not occur in ee genotype dogs. The most dominant allele in this group is thought to be aY, which causes sable and fawn coloration of the coat. Sometimes the reddish hair has black tips. Some examples of this type of dog are sable Collies and Shetland Sheepdogs as well as fawn Great Danes and Pugs. The next allele in dominance is aW, which is characterized by alternately banded hair and sometimes is called “wild type”. This type causes hair to change its color during growth from light to dark resulting in bands as seen, for example, in some German Shepherds or wolves.
The black-and-tan allele, at, gives a black dog its tan markings. This is typical of many hounds (Afghan Hound, Dachshund, Saluki) as well as Rottweilers and Doberman Pinschers. The same allele is responsible for tri-color dogs (black-and-tan with white), for example in Shetland Sheepdogs. The last allele is a recessive black “a”, which is considered to be recessive to all other allele in the A group. Such aa genotype dogs are black. This can be seen in German Shepherds and Shetland Sheepdogs (bi-color).
There are other groups of genes responsible for coat coloration. They are C (albino), D (blue dilution), G (graying), M (merle), R (roaming), S (white spotting), T (ticking), and K (dominant black). The work of identifying them is still in progress. HeathGene Laboratory would like to thank Dr. Sheila Schmutz from the University of Saskatchewan (Canada) for her dedication and support in this research
Canine Coat and Nose Color Testing
ORANGE e / e B/b D/d AND C/C ( BUTTERCUP + SABLE )
CREAM e / e b/b d/d AND C/C
CREAMY WHITE E / e b/b d/d AND C/c ( CREAMY AND ICE CREAM)
ICE WHITE E / e b/b d/d AND c/c
eeBB | red/cream/orange | black | none |
eeBb | red/cream/orange | black | brown |
eebb | red/cream/orange | brown | brown |
Canine Color Genetics
BY
Dogs have a wide variety of genes that influence color. Further, the same genes may give a very different effect on different types and lengths of coats. While this site is primarily concerned with Shetland Sheepdog colors and a long, working-type (double) coat, I will use comparisons from other breeds and even other species whenever it seems useful. References, including other mammalian color genetics, are on a separate page.
One of the biggest problems people have with genetics is the assumption that a defined trait - size, ear type, color, yappiness - is due to a single gene. In fact, genes code for two types of things. One, which is relatively well understood, is the structure of a particular protein. The normal equivalent of the albino gene, for instance, codes for tyrosinase, an enzyme which breaks up the amino acid tyrosine as a first step in producing melanin, the major pigment in mammalian skin and hair. In an albino, this enzyme cannot be produced, and as a result melanin cannot be produced. A second type of gene controls when and where other genes are turned on or off. These genes are the subject of vigorous ongoing study, and probably have a major impact on such things on the number of vertebrae in the spine or the age at which growth is complete. I've included a page which defines some of the terms used in genetics, as well as explaining dominant, recessive and incompletely dominant genes. Right now, let's look at some of the gene series (loci) known to influence canine color, and try to get a feel for what they do.
Before starting our list, we need to know that mammals have two forms of melanin in their coats. One, eumelanin, is dark, though it can vary somewhat in color due to variations in the protein that forms the framework of the pigment granule. The base form of melanin is black. Melanin can also appear brown (often called liver, chocolat or red in dogs) or blue-gray. The second pigment, which varies from pale cream through shades of yellow, tan and red to mahogany (as in the Irish Setter), is called phaeomelanin. There are at least three and possibly as many as four gene series that determine where, on the dog and along the length of the hair, eumelanin and phaeomelanin appear.
The generally recognised color series (loci) in dogs are called A (agouti), B (brown), C (albino series which may not be in dogs at all), D (blue dilution) E (extension), G (graying), K (dominant black or brindle), M (merle), R (roaning), S (white spotting) and T (ticking.) Some of these gene series are controversial; others have been confimed to correspond to particular stretches of DNA. There may be more, unrecognised gene series, and in a given breed modifying factors may drastically affect the actual appearance. Thus one school of thought holds that the round spots on a Dalmation are due to the same gene that produces the roaned areas on a German Shorthair Pointer, but with vastly different modifiers.
A, the agouti series. The standard assumption, based on Little's research, was that this series contained four alleles (different forms of the gene). One of these, dominant black, has now been shown not to be at the A locus at all, but at the new locus K (for the last letter in black.) A fifth allele has now been shown to exist in Shetland Sheepdogs, German Shepherd dogs, and several other breeds, and a sixthmay exist in certain "saddle-tan" breeds.
- According to Little, As produced black without any tan on the dog. (White markings are due to a different gene, and there are other genes that can modify the black to brown or blue dilute. Note that the agouti series is known in a number of mammals, and dominant black is almost always found in a different series. Dominant black has now been removed from the A locus
- Ay produces a dog which is predominantly tan (phaeomelanin) sometimes with black tipped hairs or interspersed black hairs. The usual term for this color in dogs is "sable." In examining dogs from Ay breeds, I have generally found that even if there is no other black on the coat, the whiskers (the course, stiff vibrissae on the muzzle and over the eyes, not the "beard" seen with some terrier coats) are black if they originate in a pigmented area. Examples of Ay dogs include Collies, fawn Boxers and Great Danes, and some reds (Basenji red is thought to be Ay, for instance.) Ay is incompletely dominant to at. That is, an Ayat dog is on average darker (more black hairs) than an AyAy dog, but the difference is generally within the range of color for AyAy within the breed.
- at, present in double dose, produces a dog which is predominantly black, with tan markings on the muzzle, over the eyes, on the chest, legs, and under the tail. A Dobermann or Rottweiler is a good example of the classic black and tan pattern. The Bernese Mountain Dog shows the effect of black and tan combined with white markings, often called tricolor.
- aw is the fourth allele considered by Little. This is the wild "wolf-color" seen in Norwegian Elkhounds and possibly in some salt-and pepper breeds. It differs from sable in two ways. First, the tan is replaced by a pale cream to pale gray color. Second, the hairs are normally banded - not just the scattering of black-tipped hairs sometimes seen in a sable, but several bands of alternating light and black pigment along the length of the hair. Little was unable to determine the dominance relationship of this gene, or even to say with certainty that the banding and the reduction of tan pigment were due to the same gene.
Although Little did not make any distinction between the Dobermann black and tan and the "saddle tan" seen in many terrier breeds (black "saddle" but extensive tan on legs and head), it seems possible that a fifth gene exists in the A series. For the moment I'll call it "saddle tan," asa. It seems recessive to Ay sable, but other dominance relationships in the series need more investigation. This would be the gene responsible for the "red-headed tri" seem in Welsh Corgis, and in that breed the dominance relationship appears to be Ay dominant to asa dominant to at.
Finally, at least two breeds (Shetland Sheepdog and German Shepherd) have a fully recessive black as the only black. Since black is the bottom recessive of the A series in many other mammals, it seems logical to assign this color to recessive black, a, and state that recessive black is caused by aa at the agouti locus. This has in fact now been confirmed by DNA analysis. The gene a has also been found in other breeds in which most blacks are due to the K locus. At the present time, it is known to occur mostly in herding and Spitz breeds. Sheila Schmutz summarizes the DNA studies.
An interesting possibility is that aa may actually prevent the formation of phaeomelanin pigment. If this is the case, aaee dogs should be white--and this combination has actually been found in some Samoyeds.
B, the brown series. This series is relatively simple. B, in single or double dose, allows the production of black pigment. A bb dog produces brown pigment wherever the dog would otherwise have produced black. The gene apparently codes for one of the proteins that makes up the eumelanin pigment granule, so the bb granules are smaller and rounder in shape as well as appearing a lighter color than those of a dog carrying B. This gene is responsible for a number of liver and chocolate colors, especially in the sporting breeds. The same gene produces some "reds" (in Australian Shepherds, Border Collies, and Dobermanns, for example), and probably the bronze Newfoundland. It has some effect on the iris of the eye and on the skin color, including the eye rims and the nose leather. Phaeomelanin (tan) is very little affected, so the color of the tan points on a red Dobermann (atatbb), for instance, is little affected. I have seen little discussion of the effect of brown on a sable dog, but I would expect a brown nose leather and eye rims, with the coat shaded brown rather than black. Probably the dog would closely resemble a sable, perhaps with an orangey cast and a light nose. Note that some shades of liver, though a eumelanin pigment, overlap some shades of tan, a phaeomelanin pigment. In particular the deadgrass color (bbcchcch) can overlap recessive yellow (ee)
C, the albino series. This again is a fairly complex locus, especially in other mammals. The top dominant, C, allows full color to develop, and is probably the structural gene for tyrosinase. The bottom recessive, c, does not appear to occur in dogs, but in other mammals it completely prevents the formation of any melenin in the coat or the irises of the eyes, giving a pink-eyed or red-eyed white. It is worth pointing out that human albinos from dark-skinned parents often show some yellowish or reddish hair and even skin color, but it seems this is not due to granular melenin. c, therefore, is a form of tyrosinase which cannot act as it is intended to in the formation of melanin. Since c is simply a non-working form, there may be more than one form of c gene (lots of ways to get something not to work), and there is some evidence that when two different forms are mated, colored offspring may result.
There are a number of intermediate genes where the mutation apparently produces a partly active form of tyrosinase. Some C alleles known in other mammals are:
- C full color, allows full expression of whatever pigment is prescribed by other genes. Most dogs are CC.
- cch, chinchilla or silver, when present in double dose removes most or all of the phaeomelanin pigment with only a slight effect on black pigment. This is named after a small fur-bearing South American rodent called the chinchilla.
- ce, extreme dilution, has also been proposed for the dog, but without evidence.
- ch, Himalyan, is not known to occur in the dog. In homozygous form, it makes the formation of eumelanin dependant on the temperature of the skin. Thus a genetically solid black animal will have reduced black on the extremities (seal brown) and an almost white color on the body. The effect on tan/orange pigment is confusing - the tan in agouti hairs is removed, but that resulting from the orange gene in cats (not in dogs) remains intense on the extremities. There is reason to suspect that this gene, as well as some forms of chinchilla, also affects the organization of the brain, particularly in the neural pathways from the eyes to the brain. There may be a reason for Siamese cats to be cross-eyed. Eyes are normally blue or pink.
- cp, platinum, is optically similar to albino but retains very slight tysonase activity and in the mouse is described as retaining some luster in the coat as opposed to the pure white seen in albino. At one time I hypothesized that the white Doberman, with pale blue eyes and pink nose, was due to a homologous gene, but there is no evidence that white in this breed is due to abnormal tyrosinase.
- c, albino, is not known to occur in the dog as a regular part of any breed color, though possible candidates for mutations to c have been recorded. As mentioned above, the c gene cannot produce working tyrosinase, and a cc individual cannot produce melanin pigment.
As seen from the above, C is known to have a number of different forms and effects. Dogs do have genes that lighten phaeomelanin more than eumelanin, but they are apparently not at the C locus. They are sumtimes lumped as rufous genes, making phaeomelanin more or less intense, as is seen in the variation in color between the Irish Setter and a very light yellow lab.
The usual assumption is that dogs have at least one mutant allele, cch which when homozygous lightens phaeomelanin (yellow) pigment to cream and more weakly affects liver and longhaired black. A second proposed allele, ce may be responsible for further reduction of cream to white in some breeds, or modifying alleles may be responsible for the further lightening in these cases. Although it is now fairly certain that these are not at the C locus, something does affect phaeomelanin pigment, and for the moment I will keep the c nomenclature.
Although C in other mammals appears to be fully dominant over any of the other alleles, the dominance relationship between the others generally goes in the direction of more color incompletely dominant over less color, the heterozygote generally resembling but not necessarily identical to the homozygote with more pigment
D, the dilution series. This, again, is a relatively simple series, containing D (dominant, full pigmentation) and d (recessive, dilute pigment). In contrast to C, which has its strongest effect on phaeomelanin, or B, which effects only eumelanin, D affects both eumelanin and phaeomelanin pigment. It is thought to act by causing the clumping of pigment granules in the hair. Like B, it often affects skin and eye color, and in some breeds dd has been associated with skin problems. "Blue" is the term most often used to describe dd blacks. If a solid liver dog also is dd, the result is the silvery color seen in Weimararners and known as "fawn" in Dobermans. (In most breeds, fawn refers to Ay yellows.)
While dd acting on black or liver is a part of the genotype of several breeds, dd acting on sable is relatively rare. For one thing, the action of dd on phaeomelanin has been described as a flattening or dulling of color. The cinnamon color in Chows is probably due to an AyAydd genotype, but otherwise the combination of dd with phaeomelanin coat color seems limited to breeds in which color is of little importance (e.g., blue brindle in Whippets.)
Although D is usually described as completely dominant to d, I have seen one blue merle Sheltie bitch who suggested that this may not always be the case. The black merling patches in this bitch were actually an extremely dark blue-gray. Other than this she was an excellently colored blue merle. The owner insisted that she was not a blue, but that she had relatives who were. I suspect that this bitch may have been Dd, with the additional diluting effect of the merle gene allowing the normally hidden effect of a single dose of d to show through.
E, the extension series. This series, like A, has undergone considerable modification from Little's description. In most mammals, the E series includes Ed (dominant black), E (normal extension) and e (recessive red or yellow, and sometimes some intermediate alleles called Japanese brindles. In dogs, this is clearly not the case; breeding experiments have conclusively proven that dominant black and recessive red are not in the same series. This has led to dominant black being thrust into the A series, which as already mentioned conflicts with results in other mammals, and has since been resolved by putting dominant black into a new locus, K, not recognized by Little.
In this summary, I will give the genes as postulated by Little, followed by a brief discussion of what DNA studies have shown. Note that the question was never whether the genes occur, but whether they were in fact alleles in the same gene series. With regard to e and E, recent sequencing of the e and E genes in dogs show definite homology with those in other species.
- Em, mask factor. This gene replaces phaeomelanin (tan) with eumelanin (black) over part of the dog. There is considerable variation in the area of replacement, probably affected by modifiers but possibly involving more than one form of Em. At its weakest the mask factor may produce black hair fringing the mouth, or a slightly smutty muzzle. At its strongest (Belgian Tervuren) most of the head is black, and there is considerable blackening of chest and legs. The effect of Em shows to its fullest extent on clear sable dogs (AyAy), but is visible on the tan points of black and tan dogs (atat) as well. In its strongest version, it can change a black and tan to a pseudo-black, with tan so restricted in its distribution that it may not be immediately apparent that the dog is not black. The occasional "black" puppy produced by two Tervuren parents may be this type of black, with two AyatEmEm parents producing an atatEmEm puppy, but aa can also occur in this breed. A similar but not quite as strong blackening of the head of a genetic black and tan occurs in German Shepherds.
- Ebr, brindle. This gene probably got into the E series by mistaken homology with Japanese brindle, which behaves quite differently from brindle in the dog. In Japanese brindle, the patchy color is believed to be due to two alleles of the E series side by side on the same chromosome. Only one can be expressed, and different parts of the animal will show the expression of different genes. The result is a coat made up of random small patches of tan and black pigment, rather like a tortoiseshell cat. If a Japanese brindle animal also has the genes for extensive white spotting, the tan and black pigmented areas tend to become larger and more compact, similar to what one sees in a calico cat (genetically, a tortoiseshell with white markings.) There is a canid which might be Japanese brindle with white spotting, the Cape hunting dog, Lycaon pictus. This animal has a coat which is a rather random patchwork of black, yellow and white. The color has very little similarity to brindle in the dog.
Brindle in dogs consists of black, vertical stripes on a sable/fawn background, usually rather soft-edged, but much more regular that a typical Japanese brindle, and showing no tendency for the tan and black patches to become more distinct in the presense of white spotting genes. Genes that affect eumelanin will affect the dark stripes, so a bb brindle, for instance, will have brown rather than black stripes. Brindle on a black and tan will show only in the tan areas, while brindle on a black cannot be distinguished at all. DNA testing has confirmed that brindle in the dog is in fact not at the E locus, but rather an allele of the K locus, unrecognized by Little.- E, normal extension of black, allows the A-series alleles to show through with no masking or brindling. It is apparently recessive to Em.
- e, recessive red, overrides whatever genes is present at the A or K loci to produce a dog which shows only phaeomelanin pigment in the coat. Skin and eye color show apparently normal eumelanin, although some ee dogs appear to show reduced pigment on the nose, especially in winter (snow nose.) A number of breeds show recessive red as a normal or even breed-wide characteristic - Irish Setters, Golden Retrievers, yellow Labradors. In a few breeds such as the Cocker Spaniel "reds" may be either AyAy or ee, and crossing the two can produce unexpected blacks. I believe there may be a key in the color of the whiskers, which on my observations seem to be black in AyAy breeds and straw to cream (dilute red) in ee breeds, always assuming the whisker base sprouts from a pigmented area. Little hypothesized that dogs with both forms of red (Ay-ee) were not viable and would be lost before birth.
G, the graying series. Although only two genes were recognised in this series by Little, this may be a more complex locus, or genes that affect graying may reside at more than one locus. The effect of G, in single or double dose, is the replacement of colored by uncolored hairs as the animal ages, very much like premature graying in human beings. This gene should be suspected in any breed where a dark puppy pales and washes out with age, and the paling is due to interspersed white hairs. The gene is almost certainly present in some Poodles, Old English Sheepdogs, and terriers. The fading may start immediately after birth or after a period of weeks to months has elapsed, and may go as far as it is going to by the first adult coat or may continue through the animal's lifetime. G may or may not be the gene involved in the graying of muzzle and over the eyes in aged dogs, or in the lightening of black to steel blue without interspersed white hairs. This is a series that definitely needs more work.
K, named for the last letter of blacK. This series is a new one as far as the genetic literature is concerned, but is now well confirmed by DNA analysis. It includes three members: Dominant black KB, mask kbr, and "yellow" ky, in that order of dominance. The actual color of the dog will depend also on the genes carried at the A and E loci.
- KB, dominant black. If this gene is present and the dog is capable of getting eumelanin into the coat, the dog will be black. As only ee appears capable of keeping eumalanin out of the coat, this means that a dominant black dog must have either E or Em in its genetic makeup. Most black dogs are of this type, as a is rare in most breeds. KB will hide whatever is present at the A locus.
- kbr, brindle. If this gene is present along with E or Em but KB is not, whatever parts of the coat that would otherwise be tan will show soft-edged eumelanin stripes on the phaeomelanin areas. At one extreme the pattern may consit of a few dark hairs in vertical lines on an otherwise tan background, at the other the dog may be almost all eumelanin with faint tan stripes. The A locus will still determine the overall position of eumelanin and phaeomelanin in the coat, but the phaeomelanin areas will now be striped with eumelanin. If aa or ee are present kbr cannot be detected even if it is present.
- ky, yellow. If only this gene is present at the K locus (kyky) the color of the dog will be determined by the genes at other loci.
M, merle. This is another dilution gene, but instead of diluting the whole coat it causes a patchy dilution, with a black coat becoming gray patched with black. Liver becomes dilute red patched with liver, while sable merles can be distinguished from sables with varying amounts of difficulty. The merling is reportedly clearly visible at birth, but may fade to little more than a possible slight mottling of ear tips as an adult. Merling on the tan points of a merled black and tan is not immediately obvious, either, though it does show if mask factor is present, and may be discernable under a microscope. Eyes of an Mm dog are sometimes blue or merled (brown and blue segments in the eye.)
Although merle is generally treated as a dominant gene, it is in fact an incomplete dominant or a gene with intermediate expression. An mm dog is normal color (no merling). A Mm dog is merled. But an MM dog has much more white than is normal for the breed (almost all white in Shelties) and may have hearing loss, vision problems including small or missing eyes, and possible infertility (Little). The health effects seem worse if a gene for white markings is also present. Thus the dachsund, which is normally lacking white markings, has dapples (Mm) and double dapples (MM) the latter often having considerable white, but according to Little other effects are limited to smaller than normal eyes. In Shelties, Collies, Border Collies, and Australian Shepherds, all of which normally have fairly extensive white markings, the MM white has a strong probability of being deaf or blind. The same is probably true with double merle Foxhounds and double merles from Harlequin Great Danes with the desired white chest. A few double merles of good quality have been kept and bred from, as a MM double merle to mm black breeding is the only one that will produce 100% merles.
It is possible that merle is a "fragile" gene, with M having a relatively high probability of mutating back to m. The observed pattern would then be the result of some clones of melanocytes having suffered such a back mutaion to mm while they are migrating to their final site in the skin, producing the black patches, while others remained Mm. This hypothesis also explains why a double merle to black breeding occasionally produces a black puppy, the proposed back mutation in this case occurring in a germ cell. On the other hand, the observed blacks from this ype of breeding may actually be cryptic merles - genetically Mm, but with the random black patches covering virtually all of the coat.
The merle gene has now been sequenced, though no commercial test is available.
Merle is a part of the pattern of ragged black spots seen in the harlequin Great Dane. There appears to be an additional gene which removes the dilute pigment, leaving the "blue" area clear white. The fact that harlequins continue to produce merles argues that animals pure for this proposed extra factor may not exist, and one possibility is that a homozygote for this whitening factor is an embryonic lethal. Interestingly, there are recent reports of Shelties born with a harlequin pattern, but in this case the "blue" area actually develops color with time, winding up a light silvery blue. These dogs appear to have larger than normal black areas, at the extreme being so-called cryptic merles, that is, no blue is visible without an extensive search. Other shelties born harlequin or "domino" retain the white body color.
Although Danes are usually solid color, the harlequin color description includes a preference for a white neck and front. Since the black patching is as apt to be on neck and front as anywhere else, this requires incorporation of a gene for white spotting (probably irish spotting, si si). Given that SS double merles seem to fare better than their si si counterparts, I would expect that double merles from harlequin Danes with patched fronts and necks might be healthier than from those that fit the standard better. The harlequin description also faults black hairs in the white area. The harlequin - silver blue pattern in Shelties could be an extreme case of black hairs in the white area. Both harlequins and the silver-blue merle Shelties have occasional patches of gray (merle?) as well as black, though this is not considered desirable.
It should be emphasized that merle cannot be detected on an ee yellow. In breeds which have both colors (Pomeranians and Chihuahuas come to mind) merles should never be bred to yellows, as the yellow may be carrying the merle gene invisibly.
R, roan. This may or may not be a true series. Both Little and Searle suggest that roan may simply be a very fine ticking, with dark hairs growing in an initially white area of the coat. A second type of roan, in which white hairs develop in an initially dark coat, could be due to gray or could be a type of roaning different from the progressive development of dark hair in a light area. In any event, roan (R) appears to be dominant to non-roan (rr). It is not clear whether this is full dominance or incomplete dominance. I will here treat roan as being at the ticking locus.
S, white spotting. This is another somewhat unsatisfactory series, and one in which modifying genes appear to have a very large effect. Certainly there are genes for solid color, for a more regular white spotting, and for basically white with some colored markings. But the variability within each type makes it unclear how many alleles actually occur at this locus or even whether more than one loci are involved. In general dominance is incomplete, with more color being dominant over less color. Heterozygotes commonly resemble the more-pigmented homozygote, but with somewhat more white. I will give the usual outline below, but recognize that this is subject to change.
- S, solid color. This is the normal gene in breeds without white markings. An SS dog can completely lack white, but it can also express very minor white markings - white toes, white tail tip, or a star or streak on the chest. SS breeds generally fault these markings.
- si, irish spotting. Irish spotting is generally confined to the neck, the chest, the underbody, the legs and the tail tip. White does not cross the back between the withers and the tail, though it may appear on the back of the neck. Breeds with "Collie markings" which breed true for the markings are generally si si.
- sp, piebald. This is a more difficult gene to identify. Certainly some breeds, such as parti-color Cockers, seem to breed true for piebald. Crosses of parti-color and solid in Cockers, however, often have minor white marking. Piebald and irish spotting seem to overlap in phenotype in one direction, while piebald and extreme white overlap in the other. In general, it seems a piebald has more than 50% white, white often crosses the back, and the pattern gives the impression of fairly large colored spots on a white ground.
- sw, extreme white piebald. Extreme white piebalds range from the color-headed whites (Collies, Shelties) which may also have a few colored spots on the body, especially near the tail, through dogs with color confined to the area around the ear or eye (Sealyham, White Bull Terrier, Great Pynenees) to some pure whites (Dalmation ideal). There is some anecdotal evidence that swsw dogs without color on or near the ear have a higher probability of deafness than dogs with color on the ears, but this varies with breed and it is not known whether a separate allele of S might be involved. In Boxers, some whites are produced from show-marked parents. Little believed that the Boxer lacked the gene for si, the irish-type spotting desired in the show ring being produced by heterozygosity for S and sw. Since the Boxer club is adamantly opposed to any breeding of whites, even test breeding, this has not been independantly confirmed.
All of the spotting genes are assumed to be affected by the action of modifiers, with + (plus) modifiers being generally understood to increase the amount of pigment (decrease white) while - (minus) modifiers being assumed to decrease the amount of pigment (increase white.) Merle appears to act as a minus modifier, in addition to its effects on coat color.
It is not clear to what extent the S series affects head pigment. Color-headed white shelties, for instance (swsw), can have completely colored heads - not even a forehead star or white nose. On the other hand, relatively conservatively marked dogs can appear with half white or all white heads. There is probably at least one other gene series that affects head markings. It is at least possible that the plus and minus modifiers affect head and body markings simultaneously.
T, ticking. Some dogs develop flecks of color in areas left white by genes in the S series. The clearest and most obvious ticking is seen in Dalmations, where additional modifier genes have enlarged and rounded the ticks. A large number of irish, piebald and extreme white breeds also have variable ticking, though not often as obvious as the Dalmation. The color of the ticking seems to be the color the coat would be in that area if the white spotting genes were not present. Thus a genetically black and tan Dalmation (a fault) will have tan spots where a black and tan would have tan markings. A ticked sable, ayayTT or ayayTt, may not have obvious ticking, becasue there is not much contrast between the tan and the white. Careful examination, however, will often show tan flecks on the legs. Ticking on a long-haired dog is also difficult to discern. The Border Collie on the front page of my site is ticked and probably sisw, as well as having the gene(?) for half white head. The tick marks in her ruff are not visible in the photo, but they are present (if difficult to find) on the living dog.
The usual dominance relationship given is that T (ticking) is dominant over t (lack of ticking.) Some breed-specific sources suggest that ticking acts as a recessive. I am inclined to suspect incomplete dominance of T. In Border Collies, for instance, a color called blue mottle is in fact a very heavily ticked piebald. The dam of the Border Collie mentioned above was such a blue mottle, presumably TT, while Dot is apparently Tt.
Ticking is also very much affected by genes which modify the size, shape and density of tick marks. In fact roan, which can develop by the gradual growth of pigmented hair in white areas of the coat, may simply be a form of ticking.
Canine Coat Color
VetGen DNA CHROMAGENE Coat Color Testing
For many years geneticists and breeders have been aware of several locations on the chromosomes, or loci, which are responsible for the color patterns we see in dogs and other mammals. As with all genetic traits, every animal inherits one copy of each locus from each of its parents. Each of these loci is responsible for one or more traits either independently, or in conjunction with another locus. All of them act on the pathways that produce the two major pigments, phaeomelanin and eumelanin, or affect the distribution of those pigments.
The combined effect of all these loci (Agouti, Brown, Extension, etc.) is the color of the dog. Due to the complex interactions of these genes, it is possible for dogs to carry hidden colors which may appear in their offspring. Over the past several years, scientists at VetGen and elsewhere have determined the actual genes associated with many of these loci, and identified the mutations responsible for the different versions (alleles) of these genes. The fruit of this work are the tests available for many of the common coat colors and traits.
The diagram below illustrates the relationships among the major loci (A, B, E and K) involved in determining coat color. The sections that follow describe the role these loci and others play in the coat color tests that VetGen offers. These tests demonstrate that while a dog may exhibit certain color or colors, it may also be carrying other hidden colors in its genetic code that can show up in later generations.
How do the A, B, E, and K loci affect each other in determining coat color?
The accompanying graphic helps to illustrate the interactions of the genes at these four loci in a hierarchy in terms of their role in coat color. If a circle is filled with color, it means the color of the dog has been determined at that point. If a circle is still white, it means information about an additional gene is required.
The first locus to look at is the E locus. The gene at this locus is responsible for black masks when present as well as most shades of yellow and red. Any dog that is "ee" will be some shade of yellow to red, and everything happening at the A, B, and K loci will be hidden until the next generation. If the dog has any E or Em alleles, then it will not be yellow and we must look next at the K locus.
There are three versions, or alleles, of the K locus: KB, kbr, and ky. If a dog has even a single copy of KB (KBKB, KBky, KBkbr) it will be solid colored in the pigmented areas, and we go directly to the B locus to determine color. Everything happening at the A locus in these dogs is hidden until the next generation. If a dog is kyky, it will not be brindled, and we go next to the A locus to see which alleles are expressed. If a dog is kbrkbr, or kbrky, it will nearly always be brindled and we look next at the A locus to see the background color and pattern of that brindling.
The A locus has at least four alleles. There are direct tests available for Ay (fawn or sable),"a" (recessive black), and "at" (tan points). There is no direct test for the Wild type (think wolf pattern) allele which is designated a .Any dog which has at least one copy of Ay (and no KB) will be fawn or sable, either with or without brindling.Any dog that is "aa" (and no KB) will be black. Any dog that is atat or ata (and no B) will have tan points, either with or without brindling.
The next stop is the B locus. Any dog which is "bb" will be have brown fur in those areas that would otherwise be black. This holds true for both solid colored and agouti-patterned animals.
The D locus (not shown in the diagram) can alter the intensity of pigment. Animals which are "dd" exhibit grey or blue fur in place of black, and light tan or "Isabella" in place of brown. This tan is similar to some AY shades but lacking any banding or black tips on individual hairs.
AVAILABLE TESTS
E locus
The E locus is responsible for the black mask seen in many breeds, and more significantly, for the presence of the yellow to red coats of many dogs. The gene involved is known as MC1-R, which has at least three versions affecting the appearance of the dog, E, Em, and e. Dogs with two copies of e will be yellow, orange or red in their pigmented coat regardless of their genotype at all the other loci
Research at VetGen and independently at the University of Saskatchewan has identified two new alleles in the E locus, Eg and Eh. These mutations are responsible for a reverse mask or widow's peak appearance in the "domino" Afghan Hound and "grizzle" Saluki (Eg), as well as the "sable" English Cocker Spaniel (Eh). Research continues to determine if they are responsible for similar appearance in additional breeds.
Test for "e"
Analysis proves absence or presence of the mutation typically responsible for yellow, lemon, red, cream, apricot and some white in at least the following breeds and all dogs with these breeds in their lineage:
Afghan, Australian Cattle Dog, Australian Shepherd, Border Collie, Beagle, Brittany Spaniel, Cardigan Welsh Corgi, Chinese Shar-pei, Chow Chow, Cocker Spaniel, Dachshund, Dalmatian, Doberman Pinscher, English Cocker Spaniel, English Setter, English Springer Spaniel, Field Spaniel, Flat-Coated Retriever, Foxhound, French Bulldog, German Longhaired Pointer, German Shepherd, German Shorthaired Pointer, German Wirehaired Pointer, Irish Setter, Labrador Retriever, Lowchen, Pointer, Pomeranian, Poodle, Pudelpointer.
Test for Em
Analysis reveals whether a dog with a mask has one or two copies of this version of the extension locus. Animals with a single copy can produce offspring with or without a mask, while those with two copies will only produce masked offspring. The test may also be applied to black dogs where it may not be possible to tell if there is a mask. It may be present in the following breeds and all dogs with them in their lineage:
Afghan, Belgian Shepherd, Boxer, Bull Mastiff, Cairn Terrier, Cardigan Welsh Corgi, Chihuahua, Chinese Shar-pei, English Bulldog,German Shepherd, Great Dane, Greyhound, Lakenois, Pekinese, Pug, Saint Bernard, Saluki, Staffordshire Bull Terrier,Whippet.
Test for Eg and/or Eh
Analysis reveals the absence or presence of the mutations responsible for "grizzle" in Salukis and "domino" in Afghans (Eg) or "sable" and "dirty red" in English Cocker Spaniels (Eh).
B Locus
The B locus is responsible for the presence of brown, chocolate, or liver animals. It is also responsible for nose color. The gene associated with this locus is known as TYRP1. In breeds where the A locus does not come into play, any animal that has at least one B allele (and is not "ee"), will be black in pigmented coat. Those dogs, which have two copies of any of several b alleles will be brown. There are at least three such b alleles. Regardless of other loci, any animal with at least one B allele will have a black nose and pads, while those with any two b alleles will have a liver nose and pads.
Test for b
This test analyzes whether an animal has 0, 1 or 2 copies of the mutations typically responsible for brown, which is also known in some breeds as liver, chocolate, sedge, and less frequently, red. There are three primary "b" mutations that are responsible for nearly every liver or chocolate dog. A notable exception is the French Bulldog where in addition to these three mutations, there is a fourth cause of chocolate that has yet to be identified.
It can be present at least, but not exclusively, in the following breeds:
Australian Cattle Dog, Australian Shepherd,Bedlington Terrier, Border Collie, Brittany Spaniel,Cardigan Welsh Corgi, Chihuahua, Chinese,Shar-pei, Cocker Spaniel, Curly Coated Retriever,Dachshund, Dalmatian, Doberman Pinscher,English Cocker Spaniel, English Setter,English Springer Spaniel,Field Spaniel, Flat-Coated Retriever, Fox Terrier, French Bulldog,German Longhaired Pointer, German Shorthaired Pointer, German Wirehaired Pointer,Labrador Retriever, Lowchen,Miniature Pinscher,Newfoundland Pointer, Pomeranian, Poodle, Portuguese Water Dog, Pudelpointer,Scottish Terrier, Skye Terrier,Weimaraner, Wirehaired Pointing Griffon.
Also:
Any dogs that contain these breeds in their lineage.
K Locus
The K locus plays a pivotal role in coat color. This locus is a relative newcomer in our understanding of canine color, and includes traits formerly attributed by some to other genes.
The dominant allele in the series is KB, which is responsible for self-coloring, or solid colored fur in pigmented areas. This trait was formerly attributed to the Agouti (A) locus as AS, but recent breeding studies had shown this not to be the case.
There are two other alleles, kbr, and ky. KB is dominant to both kbr and ky, while kbr is dominant only to ky. kbr is responsible for the brindle trait and for a long time had been considered to belong in the E locus. Recent breeding studies had also shown this to be incorrect. The recessive allele, ky, allows the basic patterns of the A locus to be expressed. So too does the kbr allele, but with brindling of any tan, fawn, or tawny areas.
Any animal with at least one KB allele will be self-colored.
Any animal with at least one kbr allele, and no KB allele will be brindled on agouti background (see A locus).
Any animal with two ky alleles will show agouti patterns (see A locus).
The mutations responsible for these alleles were identified and described primarily by Sophie Candille in the laboratory of Dr. Greg Barsh at Stanford University.
Test for KB and ky
Vetgen can presently test for these two alleles. In some breeds, where no brindle is present, this represents a complete analysis of the locus. An example would be the Pug. In breeds where the breed standard disqualifies all but self-colored dogs, testing for these two alleles is once again all that is needed. Any animal with two KB alleles cannot produce anything except self-colored offspring. A prime example here is the Labrador retriever. In breeds where many variations are allowed, these tests can help predict the probability of potential litters to include fawn, sable, tawny, tan point, tricolor or recessive black puppies.
KB KB | self-colored (solid color in pigmented areas) |
KB kbr | self-colored (solid color in pigmented areas) |
KB ky | self-colored (solid color in pigmented areas) |
kbr kbr | allows A locus to express (tan point, tricolor, fawn, sable, tawny) with brindling |
kbr ky | allows A locus to express (tan point, tricolor, fawn, sable, tawny) with brindling |
ky ky | allows expression of agouti patterns without brindling |
A Locus
The A locus is responsible for a number of common coat patterns in the dog. Expression of all of them requires any combination of two ky or Kbr alleles at the K locus, and at least one E or Em allele at the E locus. The gene involved is the Agouti gene, and variations in it are responsible for fawn and sable dogs (Ay), wild type (Aw), tan points (at), and recessive black(a).
Test for Ay
Analysis proves absence or presence of the mutation typically responsible for fawn or sable. In fawn/ sable dogs this test shows if other agouti alleles are present but hidden (only one copy of Ay). It also demonstrates how many copies of this allele are hidden in dogs, which cannot express agouti types (KBKB, KBkbr, KBky, at the k locus and/or "ee" at the E locus).
Test for “a”
Analysis shows whether a black dog is black due to “recessive black,” or the more common black at the K locus. It also reveals whether a non-black animal carries “recessive black.” Examples of breeds:
German Shepherd Dog, Shetland Sheepdog, Belgians (Tervuren, Malinois,Lakenois, Groenendael).
Test for “at”
Vetgen is now offering a test for a mutation that is found in all tan point, phantom, tricolor, and "black/tan, chocolate/tan and liver/tan" dogs. This mutation, at, was identified and reported by researchers at the University of Saskatchewan.
In order to produce “at” pups, the pups need to inherit both an at and a ky or kbr allele from both parents, but no KB . In many breeds where the occasional tan point dog is viewed as unwanted, the k test is still warranted since the majority of dogs have two copies of this at mutation, but do not express it because of the presence of an overriding KB.
The “at” mutation is also found in recessive black dogs, but does not cause recessive black (a). In breeds that do not have recessive black (most breeds), this test alone will indicate the presence of “at”. In breeds where recessive black is present, “at” can be determined by using this test in conjunction with the recessive black test, or by our previous process of elimination approach of testing for Ay and recessive black.
It should also be noted that recessive black is present at a very low frequency in more breeds than we once thought (ie French Bulldogs, Poodles and Tibetan Mastiff).
Testing for this mutation along with Ay and recessive black (a) also allows for the identification of Aw alleles in those breeds where it is present.In breeds where only the Ay and at alleles are present, the A,sup>y test can be used to see if the fawn/sable dog is Ay/Ay (homozygous) or only has one Ay (heterozygous). If it only has one, the other allele must be at (ie Afghans,Collies,Cardigan Welsh Corgi, Dachshund, Norwich Terrier, Staffordshire Terrier).
In breeds where only Ay, a and at alleles are present, both the Ay test and the "a" test need to be performed. Any alleles unaccounted for by these two tests will be at. For example, if a dog is Ay/Ay both alleles are accounted for. If a fawn/sable dog only has a single Ay, then the other allele must either be an "a" or an at and this can be determined by running the recessive black ("a") test or the at test (ie Shetland Sheepdog, Belgian Shepherd (Tervuren, Malinois, Lakenois, Groenendael).
D Locus
The D locus is the primary locus associated with diluted pigment, which results in coats that would otherwise be black or brown instead showing up as gray, or blue in the case of black, and pale brown or Isabella in the case of brown. The melanophilin gene has recently been shown to be responsible, but not all of the dilute causing mutations have been identified yet.
M Locus
The M locus is responsible for the merle and double merle color patterns seen in some breeds. The mutation which causes merle in all of its forms has been identified. VetGen cannot offer a test for merle because it is patented elsewhere.
Coat Length
While it is not a color trait, the length of a dog's coat is of interest to many. It has recently been demonstrated that in many breeds, the FGF5 gene is responsible for whether a dog has a long coat (rough or fluffy), or a short (smooth) coat. The test Vetgen offers detects the presence or absence of the recessive allele that results in long coats when present in two copies, and as such allows dogs with short coats that carry a hidden "long coat" allele to be detected. In addition to the original coat length mutation, research at VetGen has identified some new mutations present in northern breeds (Akita, Chinook, Siberian Husky) that are responsible for the "woolly" long coat. These new tests are offered exclusively through VetGen.
Furnishings
Furnishings refer to the longer facial hair around the eyebrows, moustache, and beard commonly seen in many breeds, including the wirehaired breeds. Presence of furnishings is dominant to the unfurnished version of the gene, which depending on breed may also be referred to as satin, or sleek. VetGen offers a test to see if a furnished dog carries the recessive unfurnished trait, which is considered unfavorable in some breeds.
Curly
The gene responsible for curly coat has also been identified. Most breeds are fixed for either curly or not-curly, but in breeds where there is variation, dogs may now be tested to see if they carry zero, one or two copies of the curly or non-curly versions of the KRT71 gene.
Canine Color Testing- B Locus
|
|||||||
Description Because TYRP1 is only associated with eumelanin, this mutation only has an effect on dogs that are "EE" or "Ee" at the E-locus. Dogs that are "ee" only produce phaeomelanin in their coats, so a mutation at the B-locus will not have an effect on their coat color. However, eumelanin is still produced in the foot pads and noses of "ee" dogs, so the B-locus still has an effect on these areas. Dogs that are "eebb" will have a brown nose and foot pads, rather than black.
Cost
B-Allele Results:
|
|
|||||||||||||||||||||||||||||||||||||||||
Animal Genetics, Inc. |
|
||||||||||||||||||||||||||||
Animal Genetics, Inc. |
|
|||||||||
Animal Genetics, Inc. |
Canine Color Testing- K Locus
|
||||||
Description The Dominant Black gene consists of three different alleles, or variants. The first allele, which is dominant, is noted as "KB," or dominant black. The Dominant Black allele is actually a mutation that does not allow the agouti gene to be expressed. Because this mutation is dominant, a dog only needs to have one copy of the mutation to suppress the agouti locus. A dog that has one or two copies of the Dominant Black allele will only express his base coat color, which is determined by the B-Locus and E-Locus. He will not express any colors that occur from the agouti gene, such as "black and tan" or "tricolor." The second allele is known as the "brindling" allele, and is represented as "Kbr." The Kbr allele is a separate mutation that still allows the agouti gene to be expressed, however, causes brindling of the agouti patterns. The agouti gene represents several different colors, such as fawn/sable, tricolor, tan points, or recessive black.The Kbr allele is recessive to the KB allele, however, it is dominant over a third allele, Ky. Thus, for a dog to express the brindle pattern, he must be either Kbr/Kbr or Kbr/Ky. Dogs that are KB/Kbr will not appear brindle, but can still pass on that allele and potentially produce brindle offspring. The third allele is represented as "Ky." This allele allows the agouti gene to be expressed without brindling. When a dog is Ky/Ky at the K-locus, the agouti locus determines the dog's coat color. For example, a dog that is Ay/Ay at the agouti locus could be fawn/sable. If that same dog is KB/KB at the K-locus, the agouti locus will be hidden, and his coloration will be determined at the B- and E- loci. However, if that same dog is Ky/Ky at the K-locus, he will then be able to express agouti, and will be fawn/sable. At this time, there is no direct test for the "Kbr" allele, although it can generally be inferred through testing for the presence of the Dominant Black allele, as well as through phenotypes of the parents and offspring. Testing for the Dominant Black mutation determines if the dog is able to express agouti phenotypes, however, it is limited in that it will not tell you if the dog will be brindled.
Cost
KB-Allele Results:
|


Tests Offered (click color name below for more information):
MC1R (Melanistic Mask & Black/Red) |
Agouti |
Brown |
Dilute (Blue) | Dominant Black(K locus)| Piebald, Parti or Random White Spotting |Coat Length, Curl and Furnishings|
Order these tests on MyVGL
BackgroundMammals have two pigments that are the basis of hair color: eumelanin (black) and pheomelanin (red or yellow). The gene involved in the production of these pigments in many species including dogs is Melanocortin 1 Receptor (MC1R) which is also called Extension. Other genes modify these pigments to produce the variety of colors and patterns found in the domestic dog. The Brown gene, Tyrosinase-Related Protein 1 (TYRP1), is a modifier that dilutes black pigment to brown but does not affect red pigment. Other genes involved in dog coat color include Agouti (ASIP) which organizes the distribution of black and red pigments, Beta-defensin (CBD-103) which is unique to dogs and responsible for dominant black (K locus), and Dilute (MLPH) which dilutes black and red pigments. Other genes that add white patterns and dilute colors are also present in dogs but are specific to certain breeds. Below are expanded descriptions of the genetic tests offered by the Veterinary Genetics Laboratory for dog coat color.
Allow 5-10 business days for test results.
Canine DNA Coat Color Testing
DDC Veterinary offers DNA testing for coat color genotypes among several dog breeds. By identifying the color genotype of the parents, clients can more accurately predict the colors of future progeny. Breeders can use DNA testing as a tool to more effectively manage mating patterns and make more informed selection decisions.
DNA samples can be collected easily at home using buccal (cheek) swabs provided as part of a free DNA sample collection kit.
Dogs can be DNA tested at ANY age.
A detailed DNA report for each canine tested will be mailed within 10 business days of receipt of the samples. A summary of the result will be emailed the day of completion.
The cost for testing (per canine tested) is $58 (US) for the first locus test and $20 for each subsequent coat color locus tested requested at the time of sample submission. Payment can be submitted with the return samples.
Coat Color DNA Testing options include:
E Locus (DNA marker tested – R306ter)
Most often used to determine the relationship of the Black (dominant) to Yellow (recessive). The ee
genotype results in a yellow phenotype. (“Yellow” can represent a
variety of lighter colors including apricots, reds, creams, and whites)
Possible Genotypes:
- EE – does not carry yellow
- Ee – yellow carrier
- ee – yellow phenotype
B Locus (DNA markers tested – S41C, P345Pel, Q331X)
Associated with the presence of chocolate (also commonly referred to as liver or brown). The bb genotype usually results in a chocolate coat phenotype and liver noses among yellow dogs.
Possible Genotypes:
- BB – does not carry brown
- Bb – brown carrier
- Bb2 – brown carrier
- bb – brown phenotype
For example, with Labrador Retrievers there are 3 accepted coat color phenotypes (what you can see): Black, Yellow, and Chocolate. However, there are 9 possible reported genotypes (what you cannot see) for the E and B loci and therefore 81 possible breeding combinations.
- Black Coat: 4 possible genotypes- EEBB (black with no hidden colors), EeBB (black with hidden yellow), EEBb (black with hidden chocolate), EeBb (black with hidden yellow and chocolate)
- Yellow Coat: 3 possible genotypes- eeBB (yellow with hidden black), eeBb (yellow with hidden black and chocolate), eebb (yellow with hidden chocolate- dogs will exhibit liver noses)
- Chocolate Coat: 2 possible genotypes- EEbb (chocolate with no hidden colors), Eebb (chocolate with hidden yellow)
Click here to view our Coat Color Predictor Chart. (Requires the free Adobe Reader to view.)
D Locus (DNA marker tested – C.22G>A)
Associated with the dilution or lightening effect of the solid colors Black and Brown, with D being the dominant allele, the dd genotype results in the diluted effect.
Possible Genotypes:
- DD – does not carry dilute
- Dd – dilute carrier
- dd – dilute phenotype
Em Locus (DNA marker tested – M264V)
This allele allows the agouti protein to bind some of the
time and cause fawn/red pigment to be made on the body and the
melanocyte stimulating hormone to bind on the face instead. Dogs that
are black, brown or blue do not show their mask against their similar
body color. The mask is inherited as a dominant trait.
Possible Genotypes:
- EmEm – the dog is masked and has 2 copies of the dominant allele
- EmEx – the dog is masked & has 1 dominant allele & 1 recessive allele
- ExEx – the dog does not have a mask & has 2 recessive alleles
KB Locus (DNA marker – G23del)
The KB is responsible for allowing solid colors to be expressed in the dominant form of the allele (KB). In it’s homozygous recessive form (ky) allows the agouti (a-locus) colors to be expressed.
Possible Genotypes:
KBKB – 2 copies of the dominant allele
KBky – 1 copy of the dominant allele and 1 copy of the wild type
kyky – 2 copies of the wild type
ay Locus (DNA marker tested – A82S)
This agouti allele is responsible for fawn in breeds like the Pug or sable in breeds like the Collie.
Possible Genotypes:
- ayay - 2 copies of the fawn/sable allele
- ayaw - 1 fawn/sable allele and 1 non- fawn/sable allele
- awaw - 2 non-fawn/sable alleles
a Locus (DNA marker – R96C)
This agouti allele is responsible for recessive black. In the homozygous form aa, it will cause a recessive form of black coat in breeds like the German Shepherd Dog.
Possible Genotypes:
aa – 2 copies of the recessive black allele
aax – 1 copy of recessive black allele and 1 copy of the non-recessive black allele
axax – 2 copies of the non-recessive black allele
S Locus (DNA marker – MITF reference: Schmutz S,
TG Berryere and DL Dreger. MITF and white spotting in dogs: a population
study. Journal of Heredity 2009, 100(Supl 1):S66-S74.)
This allele is associated with Piebald, Parti,
or Random White Spotting among several breeds where these patterns are
observed. The inheritance and expression can vary depending on the
breed. For example, the gene is most often recessive in nature, however
in some breeds such as Collie, Great Dane, Italian Greyhound, Shetland
Sheepdog, Boxer, and Bull Terrier, an animal having 1 copy of the
piebald allele can exhibit some limited piebald expression.
Possible Genotypes:
NN – 2 copies of the non-piebald allele
N/S – 1 copy of the non-piebald allele and 1 copy of the piebald allele
S/S – 2 copies of the piebald allele
Samples can be mailed to: DDC Veterinary, One DDC Way, Fairfield, Ohio 45014
To order kits, call 1-800-625-0874, or use our online form.
You can also download our Inherited Traits Test Order Form, which you can print and mail or fax to our laboratory (513-881-4069). If you have any questions, please email us at contact@vetdnacenter.com.
If the puppy is a black merle, ( MERLE DILUTES PATCHES OF BLACK TO GREY ) the diluted patches or gray, lighten to light silver. THIS TELLS US THAT THE GREY PATCHES ARE A RESULT OF THE MERLE GENE. IF THIS DOG ALSO CARRIES THE CHINCHILLA GENE THEN THE GREY PATCHES WILL BE SILVER
BROWN
BROWN E/E b/b D/D AND C/C
BEAVER E/E b/b D/d AND C/C
LIGHT BEAVER E/E b/b d/d AND C/C
LILAC E/E b/b d/d AND C/c
WHITE CHOCOLATE E/E b/b d/d AND c/c ( PINK POINTS LIGHT EYES )
Lilac is caused primarily by a “double recessive” condition of bb at the B gene locus and dd at the Dilute gene locus. It is also possible to produce a Lilac color out of pairings of black-to-black, black to brown, brown-to-brown, black to blue and blue to brown IF the genes are paired correctly AND they both carry the recessive forms of the B and the D gene (“b” and “d”)
Keeping in mind that each puppy receives a copy of each allele from their parents.
The first listed allele is expressed, the second one is hidden or carried. If one parent is brown (b/b) [also called chocolate] the "b" allele is the ONLY one that can be copied and inherited by the puppy. So, the puppy will receive a copy of the "b" allele from one parent. If the other parent is B/B the only allele that the puppy can receive is "B". So, every puppy will be B/b -- black, carrying brown.
If one parent is B/b black, carrying brown.the puppy can receive either "B" or "b". If the other parent is also B/b black, carrying brown., the puppy can receive either "B" or "b". The puppies could be: B/B - black; B/b - black, and carrying brown; or b/b brown/chocolate.
The 'address' where the genes are located are called Locus (Loci is the plural). Each Locus contains the different genes that are responsible for encoding (telling) coat color. Some also change skin pigment (like the nose, eye rims and lips).
Each Locus, with it's genes, are important, because a breeder can either breed a certain color into their breeding program or breed it out. A breeder can either lighten or darken the coat color, also. Coat color can also prove sometimes that the sire, the person says sired the litter, couldn't genetically be the right one.
Pigment distribution patterns are controlled by the E and A Loci.
The E Locus is important because the genes from this Locus are responsible and control black (E/E or E/e) and red (e/e) color.
If a dog is the black (E/E or E/e) color, ---- as other color genes are added, the color either changes or remains black. Think of it like baking a cake. The batter would be the main ingredient and all other ingredients either change the color of the batter, or leave it the same.
If a dog is the red (e/e) color, ---- as other ingredients are added, the color remains the same (red or creme). This is what is called "homozygous recessive dominant". The red color is the red color you see on an clear dark red Pomeranian . It comes in different shades. from the lightest creme that looks almost white to the darkest clear red
The A Locus:
contains the genes that encode for sable (a^y), wolf (a^w), saddle (a^s), tan points (a^t), and recessive black
(a^a). In order for this coloration to be expressed (seen), the dog must also be "k/k". If you breed two dogs that have tan points, EVERY puppy in the litter will have tan points. If you can't see any tan points, look underneath the tail --- it's usually there before any place else on the body.
Color that is modified by diluting colors are controlled by the B ( black ) C ( chinchilla ) , D ( dilute ) , and M ( Merle) Loci. The dog will inherit all of these genes, either in the dominant or recessive form.

![]() |
![]() |
Dilution - D gene
homozygous recessive form (dd)
homozygous recessive form (dd)
This recessive gene has a diluting effect on both eumelanin and phaeomelanin. When present in the homozygous recessive form (dd) it dilutes black/brown eumelanin to blue, and red to cream.

If he is black (B/B or B/b) and gets d/d (which is the homozygous recessive form), that will dilute the black color to blue.
![]() |
lightened. If the Merle gene is added, the color will dilute to blue merle. This merle is true blue (d/d) merle and is much lighter than the black merle. In the true blue merle, all of the black patches have been diluted to blue, gray, and pewter colors (no black color is found). The nose, lips and eye rims will be also be gray.
If he is brown/chocolate (b/b) and gets D/D or D/d, his color remains brown/chocolate.
![]() |
If he is brown/chocolate (b/b) and gets d/d, then his brown is diluted to a dull, flat silvery-brown color called dilute brown (called Lilac ). The nose, lips and eye rims are a rosey-gray color.
![]() |
If two dogs are mated that are both b/b d/d (Lilac), all of the puppies will be Lilac. |
(phaeomelanin) with little or no effect on black/brown (eumelanin).
gene lightens yellow, tan or reddish phaeomelanin to cream.
phaeomelanin is effected more strongly than eumelanin and brown.
NOTE: Newer research indicates a chinchilla-like mutation occurs in dogs, although, tyrosinase activity hasn't
been shown to be connected. Therefore, some other factor may be involved and the dog chinchilla allele may not
belong in this series.
~~ "c^e" - is 'extreme dilution'. It causes tan to become almost white.
~~ "c^b" - or blue-eyed albino. This is an entirely white coat with a very small amount of residual pigment in the
eyes, giving pale blue eyes. It is also called platinum or silver. This allelic pair could be responsible for the white
coated, pink skinned, BLUE -eyed POMERANIAN ( VERY RARE )
~~ "c^c" - true pink-eyed albino.
|
usually the same color as the dog's coat.
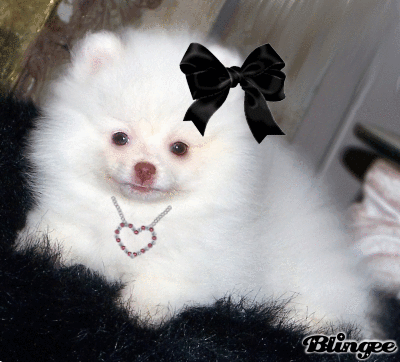
FYI:
When brown (b/b) is expressed, it means that the final step in eumelanin production has not been completed and
the pigment remains brown. The brown color is not a genetic defect.
When the alleles are in the homozygous or heterozygous dominant form of B/B or B/b, the color and pigment
(nose, eye rims and lips) remains (or directs the color to be) black.
When the alleles are in the homozygous recessive form (b/b), the color and pigment will be brown. This just means
that the final step in eumelanin production of changing brown to black did not occur. Phaemelanin (yellow/red
[e/e]) is not affected. BUT, in the e/e colored dog, if the dog is also b/b; they will be either red or yellow and will
have brown pigment (nose, eye rims and lips). e The pigment granules produced by "bb" are smaller, rounder in
shape, and appear lighter than pigment granules in "B" dogs. The iris of the eye is also lightened.
The intensity of melanin production in the coat hairs is affected by this gene. The dominant form, "C", is termed 'full
color'.
At this locus, almost all dogs are "C/C", or full color.
The lower series alleles, in order of decreasing dominance:
~~ "c^ch" - Chinchilla -- It is an incomplete dominant gene. Chinchilla lightens most or all of the red/yellow
(phaeomelanin) with little or no effect on black/brown (eumelanin). It turns black/tan to black/silver.
and red to a butter cream color.
NOTE: Newer research indicates a chinchilla-like mutation occurs in dogs, although, tyrosinase activity hasn't
been shown to be connected. Therefore, some other factor may be involved and the dog chinchilla allele may not
belong in this series. Also, there is more than one form of the chinchilla gene.
~~ "c^e" - is 'extreme dilution'. It causes tan to become almost white.
~~ "c^b" - or blue-eyed albino. This is an entirely white coat with a very small amount of residual pigment in the
eyes, giving pale blue eyes. It is also called platinum or silver. This allelic pair could be responsible for the white
coated, pink skinned, blue-eyed Pomeranians.
~~ "c^c" - true pink-eyed albino. VERY RARE in dogs.
Albino - C gene
This gene affects the intensity of melanin production in the coat hairs. The normal or dominant form, C, is what might be termed 'full color'. There are however various incompletely dominant mutant alleles postulated for this locus, with varying effects on color intensity. These mutant forms are temperature sensitive - the higher the temperature, the more effective they are (i.e, the lighter the color).
Almost all dogs are CC at this locus - full color.
The lower series alleles that have been suggested include, in order of decreasing dominance, cch, ce, cb and c.
The first, cch, is chinchilla. This lightens most or all of the phaemelanin with little or no effect on eumelanin. E.g. it turns black+tan to black+silver.
The next allele, ce, is 'extreme dilution'. Causes tan to become almost white. It is thought that the white POM might bece with another, lower, C series allele. The ce allele may be responsible for producing white hair in other breeds of dogs, like the West Highland White Terrier, while allowing full expression of dark nose and eye pigment
Moving on down we have cb, or blue-eyed albino. This is an entirely white coat but with a very small amount of residual pigment in the eyes, giving pale blue eyes. This is known as ca in cats. Can be called platinum or silver.
Finally we have c, true pink-eyed albino. ThisIS VERY RARE in dogs.
As more research is conducted in the field of(color) genetics, more information gathered and more of the'unknowns' are 'known' -Definitions:
Different terms are sometimes used for the same genetic colors, depending on breed and sometimes country too. for instance, In Pomeranians , the dilute brown, is called Beaver, Lilac or Lavender or white chocolate In Dobermans, the dilute brown, is called Isabella.etc MELANIN, AGOUTI AND RED: Melanin is the substance that gives a dog's hair its color. There are two distinct types of melanin in the dog --- eumelanin and phaeomelanin. Eumelanin is, in the absence of other modifying genes, black or dark brown. Phaeomelanin is, in its unmodified form, a yellowish color. Melanin is produced by cells called melanocytes. These are found in the skin, hair bulbs (from which the hairs grow) and other places. Melanocytes within the hair follicles cause melanin to be added to the hair as it grows. However, melanin is not added at a constant 'rate'. At the very tip of the hair, eumelanin production is usually most intense, resulting in the darker tip. A protein called the Agouti protein has a major effect on the amount of melanin injected into the growing hair. The Agouti protein causes a banding effect on the hair: it causes a fairly sudden change from the production of eumelanin (black/brown pigment) to phaeomelanin (red/yellow pigment). An example of this coloration would be like the color of a wild rabbit. The term 'Agouti' actually refers to a South American rodent that exemplifies this type of hair. | ||||||||||||||||||||||
The Extension Locus - E This refers to the extension of eumelanin over the dog's body. The dominant form, "E", is normal extension. The recessive form, "e", is non-extension. When a dog is homozygous for non-extension (e/e), its coat will be entirely red/yellow (phaeomelanin based). All dogs that have a brown (chocolate) coat will have at least one "E" allele, because of the production of eumelanin. The way to tell the difference between an Agouti red/yellow and an Extension (e/e) red/yellow dog -- is the Agouti red/yellow ( in the Pomeranian this is called Sable ) almost always have some black/brown hair in the coat (usually around the ears and tail) and the Extension (e/e) dog won't. Another way is the Agouti red/yellow must have at least one ("A^y") allele and can carry at most one other agouti allele, the Extension (e/e) can carry any two Agouti alleles (not necessarily "A^y"). DOMINANT BLACK -- "K" The dominant form of black: completely dominates all formation of phaeomelanin pigment. In the past, dominant black had been placed at the head of the Agouti series (symbol "A^s"). Now, it has been proven to be part of a separate series, the "K" series, and not at the Agouti locus at all. Dominant black (K) is epistatic to whatever is found at the Agouti locus (simply means that it causes the Agouti allele to act differently from what it normally would), however; "e/e" is dominant to "K" at the E locus. When "K" is in the dominant form, "K/K" or "K/k", there would be no expression from the A Locus and the color is dependant on what is at the E Locus. When "K" is in the homozygous recessive form "k/k", the coat color will depend on what is located on the "E" and "A" Locus. Dominant "K" codes for both dominant black and brindle in decreasing order of dominance: K -- dominant black (does not allow the A Locus alleles to be expressed) k^br -- brindle (expressed when A Locus alleles are expressed) k -- normal (allows the A Locus alleles to be expressed) A dog that is: K/K or K/k -- dominant black; dominant black carrying recessive black k^br/k^br -- brindled k^br/k -- brindled, carrying recessive black k/k -- 'normal' (recessive black) Brindling is 'stripes' of eumelanin-based (can be modified by the genes at the B and D Locus, so the color could be black, blue, chocolate or fawn) hairs in areas that are otherwise phaeomelanin based. In order to produce the brindle color, at least one parent MUST be a brindle. Brindle is dominant to its absence, so only one copy is needed. If a person has a brindle colored pup for sale and there are no brindle colors anywhere in the pedigree, then the sire that is reported on the registration papers --- genetically can not be the (true) sire. There is an exception to this if the dog is "e/e", he can be a carrier of brindle. It is thought that the three loci E, K and A act together as follows: If the dog is "e/e" at the E locus, and at the K locus, it is "K", "k^br" or "k", its coat will be entirely red/yellow (phaeomelanin based); If the dog is E/E or E/e at the E locus, and at the K locus, it is "K/K" or "K/k", its coat will be entirely dominant black (eumelanin based) [**NOTE: the phenotypic color will depend on what is at the B, D, C and M Locus]; If the dog is E/E or E/e at the E locus, and at the K locus, it is "k^br/k^br" or "k^br/k" it will be brindled with the color of the phaeomelanin part of the brindling affected by the Agouti alleles present; If the dog is E/E or E/e at the E locus, and at the K locus, it is "k/k" the distribution of eumelanin and phaeomelanin will be determined solely by the Agouti alleles present. | ||||||||||||||||||||||
The Agouti Locus - A Simply, this is how the pigment is distributed on the dog's body and hair shaft. The Agouti locus controls the formation of the Agouti protein, that in turn is one of the mechanisms that controls the replacement of eumelanin with phaeomelanin in the growing hair. The alleles of the Agouti locus can affect not just whether or not the eumelanin -- phaeomelanin shift occurs, but also where on the dog's body this happens. Two promoters are generally associated with the "wild type" version of the agouti gene.
The Cycling Promoter produces a banded hair with a black tip and yellow middle over the entire body. If only the action of this promoter is disrupted, the hair color on the dog's back will be black and its belly and inside of the legs will be yellow. This produces the black and tan color. The Ventral Promoter dictates that there will be only yellow color in the hair on the belly. The animal will have black banded hair on the dorsal (back) side and paler yellow hair on the ventral (belly) side. If only the action of this promoter is disrupted, the hair color on the dog will be banded over its entire body. This is said to be solid agouti color. If something inactivates the agouti protein, or if both promoters are disrupted, the animal will appear to be solid black. If a mutation occurs at one of these Promoters, this can cause the yellow to be expressed over most of the body. NOTE: In part of a series on Dog Coat Color Genetics by Sheila Schmutz, she states that recent studies show that the agouti signal peptide (ASIP) competes with melanocyte stimulating hormone (MSH), which produces eumelanin pigments, to bind on the melanocortin receptor and must sometimes win. Both the E allele and Em allele are responsive to agouti or melanocortin binding in dogs. However dogs that are ee have a mutation in MC1R and produce only phaeomelanin. The dog's agouti genotype doesn't affect its coat color, which will be some shade of cream, yellow or red. To further complicate things, agouti has 2 separate and somewhat distant promoters. Roughly, one seems to control ventral or belly color and the other dorsal or back color. The simplest way to "see" this is on a black and tan dog......the back is black from eumelanin pigment being made and the belly is tan or red from phaeomelanin pigment being made. The agouti gene has been mapped in the dog and DNA studies to determine which patterns are under the control of this gene in the dog are in progress. This gene undoubtedly has several alleles, but how many is still an open question. Some have been identified using DNA studies and tests for agouti phenotypes in some breeds may become available soon. Although several books attempt to state the dominance hierarchy of the agouti alleles, since no breed has all the alleles, it is not possible to know this for sure. Most books suggest that it is aw > ay > at > a. Breeding data and DNA data from our collaborative study with Dr. Greg Barsh's group at Stanford supports this. However the data confirm pairwise dominance/recessive relationships in different families.......not the entire hierarchy in one family. Decreasing in order of dominance: (**sable may be dominant over wolf in some breeds such as Pomerannians ) ~~ "a^w", 'wolf SABLE ' color - This is like "a^y" but the tan is replaced with a pale gray/cream color and the hairs usually have several bands of light and dark color, not just the black tip of sable. Example would be Keeshond, Pomeranian, Siberian and Norwegian Elkhound. ~~ "a^y", ' NORMAL sable' - also known as 'dominant yellow' or 'golden sable'. This results in an essentially red/yellow phenotype, but the hair tips are black (eumelanin). The extent of the eumelanin tip varies considerably from lighter sables (where just the ear tips are black, called "Clear Sables") to darker sables (where much of the body is dark, called "Shaded Sables"). ~~ "a^s", 'saddle' - Eumelanin is restricted to the back and side regions, somewhat like the black/tan ("a^t") allele (below). ~~ "a^t", 'tan points' - This is primarily a solid colored dog with tan (phaeomelanin) "points" above the eyes, muzzle, chest, stomach and lower legs. The hue can range from a pale biscuit to a rich ginger to a golden copper in color. Commonly seen in many breeds like Pomeranians, hounds, Dobermans, Rottweilers and Kelpies. In breeds that have the Irish spotting, along with tan points, this is known as "tri" colored (Australian Shepherds and Border Collies). ~~ "a" - last of the Agouti series is recessive black. When a dog is homozygous for recessive black (a/a), there will be no red/yellow (phaeomelanin) in its coat (unless "e/e" is present, which is epistatic to the Agouti series). Examples of breeds that show to be recessive black are German Shepherd and Shetland Sheepdog. | ||||||||||||||||||||||
BLACK or BROWN (CHOCOLATE) - B GENE LOCUS: (pigment color) This gene, when in the homozygous recessive form, has a lightening effect on eumelanin (black-based colors) only. It has no effect on phaeomelanin (red-based colors). It is believed that the Brown Locus codes for an enzyme, tyrosinase-related protein 1 (TYRP1), which catalyzes the final step in eumelanin production, changing the final intermediate brown pigment (dihydroxyindole) to black pigment. When brown (b/b) is expressed, it means that the final step in eumelanin production has not been completed and the pigment remains brown. The brown color is not a genetic defect. When the alleles are in the homozygous or heterozygous dominant form of B/B or B/b, the color and pigment (nose, eye rims and lips) remains (or directs the color to be) black. When the alleles are in the homozygous recessive form (b/b), the color and pigment will be brown. This just means that the final step in eumelanin production of changing brown to black did not occur. Phaemelanin (yellow/red [e/e]) is not affected. BUT, in the e/e colored dog, if the dog is also b/b; they will be either red or yellow and will have brown pigment (nose, eye rims and lips). e The pigment granules produced by "bb" are smaller, rounder in shape, and appear lighter than pigment granules in "B" dogs. The iris of the eye is also lightened. DILUTION - D GENE LOCUS: (dilution of pigment) Dilute Black Dilute Brown This gene has an effect on both eumelanin and phaeomelanin. When in the dominant form, "D/D" or "D/d", it allows for full color (black or red). When present in the homozygous recessive form (d/d) it dilutes black (eumelanin) to blue, and red to cream. COMBINATIONS OF B AND D IN EUMELANISTIC COATS: The effects of these 2 genes, when combined, form a range of 4 eumelanistic ('black-based') colors: The color of the pup/dog (Eumelanistic Color): B/B D/D or B/b D/d will be black in color B/B d/d or B/b d/d will be blue in color b/b D/D or b/b D/d will be brown/Chocolate b/b d/d will be flat or dull diluted brown/chocolate | ||||||||||||||||||||||
WHITE SPOTTING - S GENE: ( PARTY COLOR IN POMERANIANS ) The "S" series alleles appear to be incompletely dominant. In dogs it is thought there are four alleles that deal with white spotting: ~~ "S" - 'solid/self color'. Most dogs that are homozygous for "S/S" have no white hair at all, or possible a tiny amount, like a white tail tip. ~~ "s^i" - 'irish spotting'. This involves white spotting on most parts of the coat, but not crossing the back beyond the withers. This color pattern is evident on the Border Collie, Australian Shepherd and other breeds that have the white collar. New research has proven that the white undersides of the Border Collie is dictated by a different gene. ~~ "s^p" - 'piebald'. The white is more extensive than irish spotting, and often crosses the back. It is sometimes confused with the merle pattern. This coloration usually has large colored spots on the body. The white covers approximately 50% of the body. ~~ "s^w" - 'extreme white piebald'. A dog that is homozygous for "s^w" will be almost entirely white, like some Bull Terriers. The Australian Cattle Dog, the coloration that is called "Blue" by the ACD breeders/owners, is really the extreme piebald pattern that is also affected by the ticking gene; giving the coloration a blue appearance. This allelic pair is also responsible for the "color headed" white dogs. Often times, along with a colored head, there will also be a colored spot near the tail. | ||||||||||||||||||||||
ALBINO - C GENE: (development of pigment) The intensity of melanin production in the coat hairs is affected by this gene. The dominant form, "C", is termed 'full color'. At this locus, almost all dogs are "C/C", or full color. The lower series alleles, in order of decreasing dominance: ~~ "c^ch" - Chinchilla -- It is an incomplete dominant gene. Chinchilla lightens most or all of the red/yellow (phaeomelanin) with little or no effect on black/brown (eumelanin). It turns black/tan to black/silver. In dogs, this gene lightens yellow, tan or reddish phaeomelanin to cream. Since there is little effect on the dark eumelanin, phaeomelanin is effected more strongly than eumelanin and brown. Dilute eumelanin (blue) is effected more strongly than dark (black) eumelanin. When chinchilla is present, it dilutes brown to milk chocolate, blue to silver and red to a butter cream color. NOTE: Newer research indicates a chinchilla-like mutation occurs in dogs, although, tyrosinase activity hasn't been shown to be connected. Therefore, some other factor may be involved and the dog chinchilla allele may not belong in this series. Also, there may be more than one form of the chinchilla gene. ~~ "c^e" - is 'extreme dilution'. It causes tan to become almost white. It is thought that the white labrador might be "c^e" with another, lower, "C" series allele. The "c^e" allele may be responsible for producing white hair, while allowing full expression of dark nose and eye pigment. West Highland Terriers are thought to be e/e c^e/c^e. ~~ "c^b" - or blue-eyed albino. This is an entirely white coat with a very small amount of residual pigment in the eyes, giving pale blue eyes. It is also called platinum or silver. This allelic pair could be responsible for the white coated, pink skinned, blue-eyed Doberman's. ~~ "c^c" - true pink-eyed albino. GRAYING - G GENE: This is a dominant mutant gene that causes the dog to gray with age. The pigmented hairs are progressively replaced with unpigmented hairs. | ||||||||||||||||||||||
MERLE - M GENE: The only way a merle colored pup can be produced is if at least one parent is merle. Some breeders are of the understanding that the merle gene is a recessive gene and is carried from generation to generation. This is not correct. The merle gene is not carried, meaning -- the dog is either a merle or is not a merle. There are no exceptions to this law of genetics (for now, at least, until further research is conducted). If someone tells you that they have a litter of merled colored pups and there are no merles for many generations in their bloodlines --- then these merled pups were not sired by the sire the owner thinks there were. In fact, he should look for the hole in the fence! The merle gene is an incomplete dominant or a gene with intermediate expression and is another dilution gene. Instead of diluting the whole coat it causes a patchy dilution, with a black coat becoming gray patched with black. Brown becomes dilute brown patched with chocolate, sienna, brick, and various diluted brown colors. While sable merles can be distinguished from sables, this is sometimes very difficult because the merle coloration looks like -- to just slightly different from -- the sable color. The merling is clearly visible at birth, but may fade to little more than mottling of the ear tips as an adult. Merling on the tan points of a merle black and tan is not immediately obvious, either, though it does show if the mask factor is present. Eyes of a merle dog are sometimes blue or marbled (brown and blue segments in the eye). A "m/m" (homozygous recessive) dog is normal color (no merling). A "M/m" (heterozygous) dog is a merle. A "M/M" (homozygous dominant) dog, known as a double merle (from a merle to merle mating), has much more white than is normal for the breed and may have hearing loss, vision problems including small or missing eyes, and possible infertility. The health effects seem worse if a gene for white markings is also present. In Border Collies and Australian Shepherds, all of which normally have fairly extensive white markings, the "M/M" white has a strong probability of being deaf or blind. A "M/M", double merle, to "mm", non-merle black in color breeding, is the only one that will produce 100% merles. Cryptic or phantom (as it's sometimes called) merles are dogs which carry a merle gene but are phenotypically (look like) tri, bi or self colored. These dogs will have some small area of merling somewhere, usually a tiny patch of merle pattern on their ear, tail, top of head, etc. Keep in mind the tiny patch can be only one hair and it can be located anywhere on the body. Cryptic merles are very rare. AGAIN, a cryptic or visible merle can only be produced when one or both parents are merles. | ||||||||||||||||||||||
GENOTYPES AND COLORS: ("-" is either the dominant or recessive allele)
AGOUTI:
NON-EXTENSION RED (cream):
|
BY READING THE FOLLOWING INFORMATION
( ESPECIALLY THE INFO HIGHLIGHTED IN YELLOW )
YOU WILL GET A REALLY GOOD UNDERSTANDING OF BASIC COLOR GENETICS.
Chromosomes in a body cell are arranged in pairs, one from the SIRE and one from the DAM. Further, the code for a particular protein is always on the same place on the same chromosome. This place, or location, is called a locus (plural loci.)
There are generally a number of slightly different genes that code for forms of the same protein, and fit into the same locus. Each of these genes is called an allele.
Each locus, then, will have one allele from the mother and one from the father. How?
When an animal makes an egg or a sperm cell (gametes, collectively) the cells go through a special kind of division process, resulting in a gamete with only one copy of each chromosome. Unless two genes are very close together on the same chromosome, the selection of which allele winds up in a gamete is strictly random. Thus a dog who has one gene for black pigment and one for brown pigment may produce a gamete which has a gene for black pigment OR for brown pigment. If he's a male, 50% of the sperm cells he produces will be B (black) and 50% will be brown (b).
When the sperm cell and an egg cell get together, a new cell is created which once again has two of each chromosome in the nucleus. This implies two alleles at each locus (or, in less technical terms, two copies of each gene, one derived from the mother and one from the father,) in the offspring. The new cell will divide repeatedly and eventually create an animal ready for birth, the offspring of the two parents. How does this combination of alleles affect the offspring?
There are several ways alleles can interact. In the example above, we had two alleles, B for black and b for brown. If the animal has two copies of B, it will be black. If it has one copy of B and one of b, it will be just as black. Finally, if it has two copies of b, it will be brown, like a chocolate Labrador. In this case we refer to B as dominant to b and b as recessive to B. True dominance implies that the dog with one B and one b cannot be distinguished from the dog with two B alleles. Now, what happens when two black dogs are bred together?
We will use a diagram called a Punnett square. For our first few examples, we will stick with the B locus, in which case there are two possibilites for sperm (which we write across the top) and two for eggs (which we write along the left side. Each cell then gets the sum of the alleles in the egg and the sperm. To start out with a very simple case, assume both parents are black not carrying brown, that is, they each have two genes for black. We then have:
B | B | |
B | BB (black) | BB (black) |
B | BB (black) | BB (black) |
All of the puppies are black if both parents are BB (pure for black.
Now suppose the sire is pure for black but the dam carries a recessive gene for brown. In this case she can produce either black or brown gametes, so
B | B | |
B | BB (pure for black) | BB (pure for black) |
b | Bb (black carrying brown) | Bb (black carrying brown) |
This gives appoximately a 50% probability that any given puppy is pure for black, and a 50% probability that it is black carrying brown. All puppies appear black. We can get essentially the same diagram if the sire is black carrying brown and the dam is pure for black. Now suppose both parents are blacks carrying brown:
B | b | |
B | BB (pure for black | Bb (black carrying brown) |
b | Bb (black carrying brown) | bb (brown) |
This time we get 25% probabilty of pure for black, 50% probability of black carrying brown, and - a possible surprise if you don't realize the brown gene is present in both parents - a 25% probability that a pup will be brown. Note that only way to distinguish the pure for blacks from the blacks carrying brown is test breeding or possibly DNA testing - they all look black.
Another possible mating would be pure for black with brown:
B | B | |
b | Bb (black carrying brown) | Bb (black carrying brown) |
b | Bb (black carrying brown) | Bb (black carrying brown) |
In this case, all the puppies will be black carrying brown.
Suppose one parent is black carrying brown and the other is brown:
B | b | |
b | Bb (black carrying brown) | bb (brown) |
b | Bb (black carrying brown) | bb (brown) |
In this case, there is a 50% probability that a puppy will be black carrying brown and a 50% probability that it will be brown.
Finally, look at what happens when brown is bred to brown:
b | b | |
b | bb (brown) | bb (brown) |
b | bb (brown) | bb (brown) |
Recessive to recessive breeds true -
all of the pups will be brown.
Note that a pure for black can come out of a mating with both parents carrying brown, and that such a pure for black is just as pure for black as one from ten generations of all black parentage. THERE IS NO MIXING OF GENES. They remain intact through their various combinations, and B, for instance, will be the same B no matter how often it has been paired with brown. This, not the dominant-recessive relationship, is the real heart of Mendelian genetics.
This type of dominant-recessive inheritance is common (and at times frustrating if you are trying to breed out a recessive trait, as you can't tell by looking which pups are pure for the dominant and which have one dominant and one recessive gene.)
Note that dominant to dominant can produce recessive, but recessive to recessive can only produce recessive.
The results of a dominant to recessive breeding depends on whether the dog that looks to be the dominant carries the recessive. A dog that has one parent expressing the recessive gene, or that produces a puppy that shows the recessive gene, has to be a carrier of the recessive gene. Otherwise, you really don't know whether or not you are dealing with a carrier, bar genetic testing or test breeding.
One more bit of terminology before we move on -
an animal that has matching alleles
(BB or bb) is called homozygous.
An animal that has two different alleles at a locus (Bb) is called heterozygous.
A pure dominant-recessive relationship between alleles implies that the heterozygous state cannot be distinguished from the homozygous dominant state. This is by no means the only possibility, and in fact as DNA analysis advances, it may become rare. Even without such analysis, however, there are many loci where three phenotypes (appearances) come from two alleles. An example is merle in the dog. This is often treated as a dominant, but in fact it is a type of inheritance in which there is no clear dominant - recessive relationship. It is sometimes called overdominance, if the heterozyote is the desired state. I prefer incomplete dominance, recognising that in fact neither of the alleles is truly dominant or recessive relative to the other.
As an example, we will consider merle. Merle is a diluting gene, not really a color gene as such. If the major pigment is eumelanin, a dog with two non-merle genes (mm) is the expected color - black, liver, blue, tan-point, sable, recessive red. If the dog is Mm, it has a mosaic appearance, with random patches of the expected eumelanin pigment in full intensity against a background of diluted eumelanin. Phaeomelanin (tan) shows little visual effect, though there is a possibility that microscopic examination of the tan hair would show some effect of M. Thus a black or black tan-point dog is a blue merle, a brown or brown tan-point dog is red merle, and a sable dog issable merle, though the last color, with phaeomelanin dominating, may be indistinguishable from sable in an adult. (The effect of merle on recessive red is unknown, and I can't think of a breed that has both genes.) What makes this different from the black-brown situation is that an MM dog is far more diluted than is an Mm dog. In those breeds with white markings in the full-color state the MM dog is often almost completely white with a few diluted patches, and has a considerable probablity of being deaf, blind, and/or sterile.
ble on this site, but the gene also occurs in Australian Shepherds, Collies, Border Collies, Cardiganshire Welsh Corgis, Beaucerons(French herding breed), harlequin Great Danes, Catahoula leopard dogs, and Daschunds, at the least.
Note that both of the extremes - normal color and double merle white - breed true when mated to another of the same color, very much like the Punnett squares above for the mating of two browns or two pure for blacks. I will skip those two and go to the more interesting matings involving merles.
First, consider a merle to merle mating. Remember both parents are Mm, so we get:
M | m | |
M | MM (sublethal double merle) | Mm (merle) |
m | Mm (merle) | mm (non-merle) |
Assuming that merle is the desired color, this predicts that each pup has a 25% probability of inheriting the sublethal (and in most cases undesirable by the breed standards) MM combination, only 50% will be the desired merle color, and 25% will be acceptable full-color individuals. (In fact there is some anecdotal evidence that MM puppies make up somewhat less than 25% of the offspring of merle to merle breedings, but we'll discuss that separately.) Merle, being a heterozygous color, cannot breed true.
Merle to double merle would produce 50% double merle and is almost never done intentionally. The Punnet square for this mating is:
M | M | |
M | MM (sublethal double merle) | MM (sublethal double merle) |
m | Mm (merle) | Mm (merle) |
Merle to non-merle is the "safe" breeding, as it produces no MM individuals:
m | m | |
M | Mm (merle) | Mm (merle) |
m | mm (non-merle) | mm (non-merle) |
We get exactly the same probability of merle as in the merle to merle breeding (50%) but all of the remaining pups are acceptable full-colored individuals.
There is one other way to breed merles, which is in fact the only way to get an all-merle litter. This is to breed a double merle (MM) to a non-merle (mm). This breeding does not a use a merle as either parent, but it produces all merle puppies. (The occasional exception will be discussed elsewhere.) In this case,
M | M | |
m | Mm (merle) | Mm (merle) |
m | Mm (merle) | Mm (merle |
The problem with this breeding is that it requires the breeder to maintain a dog for breeding which in most cases cannot be shown and which may be deaf or blind. Further, in order to get that one MM dog who is fertile and of outstanding quality, a number of other MM pups will probably have been destroyed, as an MM dog, without testing for vision and hearing, is a poor prospect for a pet. In Shelties, the fact remains that several double merles have made a definite contribution to the breed. This does not change the fact that the safe breeding for a merle is to a nonmerle.
Thus far, we have concentrated on single locus genes, with two alleles to a locus. Even something as simple as coat color, however, normally involves more than one locus, and it is quite possible to have more than two alleles at a locus. What happens when two or more loci are involved in one coat color?
Basic Genetics II: Multiple Loci
Usually more than one gene locus is involved in coat color. We'll take one of the simplest, in which the two loci each have two alleles, with a simple dominant-recessive relationship. The model we will use is the Labrador Retriever. One locus we have already examined: the brown locus. We will now add a second locus, on a different chromosome, called E. An EE or Ee dog will show whatever eumelanin pigment is possible. An ee dog apparently can manufacture only phaeomelanin in the hair, though the skin and eye pigment still includes melanin (of whatever color is allowed by the B series).
A black Lab may be BBEE, BBEe, BbEE or BbEe - any combination that includes at least one B and one E gene.
A chocolate (brown) Lab may be bbEE or bbEe.
A yellow Lab with a black nose may be BBee or Bbee
A yellow Lab with a liver nose is bbee - but since ee dogs tend in many cases to lose nose pigment in winter, this may not be easy to distinguish from BBee or Bbee.
Suppose we mate two BbEe dogs, both blacks carrying brown and yellow:
BE | Be | bE | be | |
BE | BBEE (pure for black) | BBEe (black carrying yellow) | BbEE (black carrying brown) | BbEe (black carrying brown and yellow) |
Be | BBEe (black carrying yellow) | BBee (pure for yellow, black nose) | BbEe (black carrying brown and yellow) | Bbee (yellow carrying brown) |
bE | BbEE (black carrying brown) | BbEe (black carrying brown and yellow) | bbEE (pure for brown) | bbEe (brown carrying yellow) |
be | BbEe (black carrying brown and yellow) | Bbee (yellow carrying brown) | bbEe (brown carrying yellow) | bbee (brown-nosed yellow) |
Each puppy has one chance in sixteen of having the combination shown in any section of the table above. In this mating between two black dogs both carrying brown and yellow, there is a 9/16 probability that a particular pup will be black, a 3/16 probability that the pup will be brown, a 3/16 probability that the pup will be a black-nosed yellow, and a 1/16 probability of a brown-nosed yellow. Since nose color does not come into registration, the registered colors would be 9 black:3 brown:4 yellow.
What happens if more than two loci are involved? The basic principle is the same - put all of the possible combinations in a sperm cell along the top and all of the possible combinations in an egg cell along the left side. The problem is that the number of possible combinations doubles for each additional locus. For a single locus, we had a 2 x 2 square with 4 cells. For two loci, we had a 4 x 4 square with 16 cells. With three loci, we have an 8 x 8 square with 64 cells. Besides, we've pretty well exhausted the acceptable colors for Labs.
Shetland Sheepdogs might be a good model for our three-locus model. For the moment we'll omit the recessive black, and consider that Sheltie color is determined by three loci.
At the A (agouti) locus, ay is sable and at is tan-point (black and tan = referred to as tricolor if a white spotting gene is present.) An ayat dog is sable, but generally somewhat darker than an ayay dog. The difference is generally of the same order as the difference within ayay or within ayat, so it is not possible to be absolutely sure whether at is present by looking at the dog.
At the M locus, Shelties have both M and m, as discussed earlier. Mm produces blue merle in atat dogs and sable merle on ayat and ayay.
At the S (spotting) locus most correctly marked Shelties have two copies of si, Irish spotting. A sisi dog generally ranges from white on the chest and feet to high white stockings, white tail tip, and a full shawl collar. The probability of the full collar, as well as white stifles, seems to be somewhat enhanced if the dog is sisw, so sw, color headed white, tends to be maintained in the breed as well. (I am keeping it simple by ignoring sp, piebald, which may also occur in Shelties.) swsw dogs are predominantly white with color on the head and perhaps a few body spots. While healthy, they cannot be shown.
Suppose we mate two white-factored, tri-factored sable merles (not a likely mating, but this is an illustration!) The genetic formula for each parent is ayatMmsisw. There are eight possible gametes for each sex:
ayMsi | ayMsw | aymsi | aymsw | atMsi | atMsw | atmsi | atmsw | |
ayMsi | DMS | DMS | SM | SM | DMS | DMS | StM | StM |
ayMsw | DMS | DMS* | SM | WSM | DMS | DMS* | StM | WStM |
aymsi | SM | SM | S | S | StM | StM | St | St |
aymsw | SM | WSM | S | WS | StM | WStM | St | WSt |
atMsi | DMS | DMS | StM | StM | DM | DM | BM | BM |
atMsw | DMS | DMS* | StM | WStM | DM | DM* | BM | WBM |
atmsi | StM | StM | St | St | BM | BM | T | T |
atmsw | StM | WStM | St | WSt | BM | WBM | T | WT |
There is no way I could fill in this chart with the detail I used in the 2-loci charts and still have it fit readably into a browser window, so I have used a shorthand to indicate the apparent color:
- S = pure for sable with Irish markings (3)
- St = tri-factored sable with Irish markings (6)
- T = tricolor with Irish markings (3)
- SM = pure for sable merle with Irish markings (6)
- StM = tri-factored sable merle with Irish markings (12)
- BM = blue merle with Irish markings (6)
- WS = white with pure for sable head (1)
- WSt = white with trifactored sable head (2)
- WT = white with tricolor head (1)
- WSM = white with pure for sable merle head (2)
- WStM = white with tri-factored sable merle head (4)
- WBM = white with blue merle head. (2)
- DMS = homozygous merle, dilute sable markings (12)
- DM = normal homozygous merle (4)
I have not distinguished white-factored from Irish dogs, and I have ignored the possibility that the MMswsw pups (starred in chart) might not be viable. In practice such a breeding would probably never be made, as Sheltie breeders tend to avoid breeding merle to merle and white factor to white factor, but it does illustrate the variety that can be obtained with two alleles at each of three loci.
In this case, all three loci are visibly affecting the color. The only exception is the interaction between color-headed white and double merle, and this is frankly an unknown. There are times, however, when a particular gene combination at one locus can block expression of a gene combination at another locus. I will follow Searle on nomenclature and distinguish between a dominant-recessive relationship between alleles at a particular locus and an epistatic-hypostatic relationship between two loci.
The first example is very obvious, but only because the gene action is clear-cut. Consider Cocker Spaniels. They have two alleles at the S locus (S, fully colored, and sp, piebald.) An SS dog is solid color, an Ssp dog may have minor white marking (and is often unshowable) and an spsp dog is a parti-color. The second gene is ticking. Ticking works by producing flecks of color in white areas. TT produces ticks of color in any white areas on the dog, tt has clear white areas, and Tt probably produces less ticking than TT, with considerable variation among breeds. spsp and probably Ssp dogs will show ticking if T is present, since they have white areas that are "available" for ticking, though if the base color is red, tan or cream the ticking may not be obvious. But if the dog is SS, there are no white spots for the ticking to show up on. SS is thus epistatic to ticking.
The final example involves the genes for dominant black, which may or may not (my feeling is probably not, as there are records of dominant black to tricolor producing both sables and tris) be the top dominant in the A series where it is generally placed. I will assume it is at a separate locus K, with K being dominant black, epistatic to anything at the A series, while kk allows the A series to show through. We also have the E series, in which E allows the A series to show through while ee allows only red-yellow pigment in the hair. Functionally we can consider that the A locus determines where eumelanin and phaeomelanin are produced, the K locus allows only eumelanin to be produced if E is at the E locus, but ee at the E locus overrides that to allow only phaeomelanin production. Sounds like a mess? You bet it does! K at the K locus is epistatic to the A locus, but ee (pure recessive at the E locus) is epistatic to both the A and the K locus. But it agrees with what is observed.
Let's look at a breed cross between two "red" dogs. We'll take an accidental breeding I know of between a Belgian Tervuren (ayayEEkk) and a Golden Retriever (??eeKK). Note that ee is epistatic to the A series, so if dominant black is not at the A locus, we do not know what the normal A allele is in the Golden. The gametes are ayEk for the Terv and ?eK for the Golden. Every puppy inherits ay?EeKk and is black, as was in fact observed (to the initial astonishment of the owner.) If we mated two of these pups, we would get a 16/64 probability of ee which would be red regardless of what was at other loci. Of the other 48/64 (Ee and EE dogs), 75% would be Kk or KK, and hence black. so there is a 36/64 probability that a particular puppy will be black. The remaining 12/64 will show what is present at the A locus. Of the 12, we expect that 9 will have the ay gene in at least one dose, and with dominant black moved to the K locus ay is dominant over all other A alleles. So there is only a 3/64 chance that a given puppy will actually show what A allele is normal in a Golden - if in fact all Goldens have the same allele at A!
Note that in this particular case we can get identical results in the first generation by postulating a top dominant As dominant black at the A locus, with As- dogs having solid eumelanin pigmentation (unless overridden by ee.) In this case the parent gametes would be Ase and ayE, giving AsayEe black pups. In the next generation we would again get 4/16 ee red, 9/16 As-E- black, and 3/16 ayayE- sables. If we could be absolutely sure that the Terv used was not ayat, the appearance of a tricolor would be good evidence for the first hypothesis.
We still need to discuss penetrance, variable expression, and threshold traits, as well as linkage and crossing over (and their influence on the accuracy of DNA testing), test breeding, andtesting whether a suspected allele is in fact at a particular locus. Some further comments about merle are also in the works.
Basic Genetics III: Linkage and Crossing Over Up until now we have assumed that all genes were inherited independently. However, we have also said that genes are arranged on chromosomes, which are essentially long strands of DNA residing in the nucleus of the cell. This certainly opens the possibility that two otherwise unrelated genes could reside on the same chromosome. Does independent inheritance hold for these genes?
To start with, we need to consider the rather complex process that forms gametes (egg and sperm cells, each with only one copy of each chromosome) from normal cells with two copies of each chromosome., one derived from each parent. I am not going to go into the details, beyond remarking that at one stage of this process, the maternally-derived chromosome lines up with the corresponding paternally-derived chromosome, and only one of the two goes to a specific gamete. If this were all there were to it dogs, having 39 chromosome pairs, would have only 39 "genes", each of which would code for a wide variety of traits. In fact, things are a little more complicated yet, because while the paternal and maternal chromosomes are lined up, they can and do exchange segments, so that at the time they actually separate, each of the two chromosomes will most likely contain material from both parents.
At this point we need to define a couple of terms. Two genes are linked if they are close together on the same chromosome and thus tend to be inherited together. Linkage in common usage, however, may apply to a single gene having more than one effect. An example which is not linkage in the sense used here is the association between deafness and extreme white spotting. White spotting is due to the melanocytes, the cells which produce pigment, not managing to migrate to all parts of the fetus. Now it turns out that in order for the inner ear to develop properly, it must have melanocytes. If the gene producing white spotting also prevents the precursors of the melanocytes from reaching the inner ear, the result will be deafness in that ear. In other words, the same gene could easily influence both processes. Thus deafness and white spotting are associated, but they are not linked. They are due to what is called pleiotropic (affecting the whole body) effects of a single gene.
In true linkage, there is always the possibility that linked genes can cross over. Imagine each chromosome as a piece of rope, with the genes marked by colored stripes. The matching of the maternal and paternal chromosomes is more or less controlled by the colored stripes, which tend to line up. But the chromosomes are flexible. They bend and twist around each other. They are also self healing, and when both the maternal and paternal chromosomes break, they may heal onto the paired chromosome. This happens often enough that genes far apart on long chromosomes appear to be inherited independently, but if genes are close together, a break is much less likely to form between them than at some other part of the paired chromosomes.
Such breaks, called "crossing over" do occur, and occur often enough that they are used to map where genes genes are located on specific chromosomes. In general, neither linkage nor crossing over is of much importance to the average dog breeder, though one should certainly keep in mind the possibility that the spread of an undesirable gene through a breed is due to the undesirable gene being linked to a gene valued in the breed ring. Crossing over is also important in the use of marker genes for testing whether a dog carries a specific gene, most often a gene producing a health problem.
There are two distinct ways of using DNA testing to identify dogs carrying specific, undesirable genes. The first (and preferable) is actually to sequence the undesirable gene and its normal allele. This allows determination of whether the dog is homozygous normal, a heterozygous carrier, or homozygous affected. Since the genes themselves are being looked at, the results should be unambiguous. (The breeding decisions based on these results are still going to depend on the priorities of the breeders.)
In some tests, however, a marker gene is found that appears to be associated with the trait of interest, but is not actually the gene producing that trait. Such a marker is tightly linked to the gene actually causing that trait. This does not work at all badly providing that the group on which the test was validated is closely related to the group to which the test was applied. Use of this type of test on humans usually requires that the test be validated on close relatives, and applied only to people closely related to the validation group.
It is true that dogs of a given breed tend to be closely related to each other. However, the breed-wide relationship is generally through more distant ancestors than most people can trace in their own genealogy. In Shetland Sheepdogs, for instance, almost all US show stock can be traced to dogs imported from the British Isles between 1929 and 1936, with only a tiny influence of imports after 1950. This means that a crossover appearing on one side of the Atlantic since 1950 (20 or so dog generations) might not show up on the other side. Marker tests that work on U.S. populations might not work at all on British dogs, or on a dog with recent British ancestry.
Even without physical separation here is always the possibility that at some point in the breed history a crossover occurred. Quite a large fraction of the breed may have the original relationship between the marker gene and the problem gene, but if a crossover occurred in an individual who later had a considerable influence on the breed, the breed may also contain individuals in which the marker gene is associated with the opposite form of the problem gene. Since the relationship between individuals of the same breed may go back 30 generations or more, and there is a chance of a crossover occurring in each generation, linked markers need to be used with caution and with constant checking that marker test results correlate with clinical results.
Let's look more closely at this.
Let our marker gene be ma, with maa being the gene associated with the healthy gene, and mabbeing the marker that seems to be associated with the defective gene, both being true for the test population. For the genes actually producing the problem, we will use H, with Hh being the normal, healthy gene and hd being the recessive gene which causes the problem. In the original test population, maa was always on the same chromosome with Hh, and mab was on the same chromosome with hd. In other words, chromosomes are either maaHh or mabhd, never maahd or mabHh. If a dog has maa on both chromosomes, it is also Hh on both chromosomes, a genetic clear. If it has maa on one chromosome and mab on the other, it also has one Hh gene and one hd gene, and is a carrier. If it has mab on both chromosomes, it has hdon both chromosomes and is a genetic affected. At least, that is the assumption on which marker tests are based.
Now suppose that at some point a crossover occurred between the ma and H loci. The probability of a crossover may be very small in any individual breeding, but remember that there are a lot of breedings behind any particular dog. We can still assume that most of the chromosomes will still be of the maaHh or mabhd type, or the original validation of the marker test would have failed. But now suppose that a small fraction of the chromosomes are of types maahd and/or mabHh. We now have four chromosome types, and sixteen possible combinations. Some of these will test the same, since the only difference is in which chromosome comes from the mother and which from the father, but there are still sixteen possible outcomes. In the table below both the marker results (upper) and the true results (lower) are shown for each possible combination:
maaHh mabhd maahd mabHh maaHh clear maamaa carrier maamab clear maamaa carrier maamab clear HhHh carrier Hhhd carrier Hhhd clear HhHh mabhd carrier maamab affected mabmab carrier maamab affected mabmab carrier Hhhd affected hdhd affected hdhd carrier Hhhd maahd clear maamaa carrier maamab clear maamaa carrier maamab carrier Hhhd affected hdhd affected hdhd carrier Hhhd mabHh carrier maamab affected mabmab carrier maamab affected mabmab clear HhHh carrier Hhhd carrier Hhhd clear HhHh Note that in only six of the sixteen possible types is the marker indication of genotype correct. If the crossover genotypes are rare (as would normally be the case if the marker test verified at all) most of the population will be in the upper left quarter of the table, where the marker will correctly predict the true genotype. But if any of the chromosomes trace back to a crossover, a marker test may give a false sense of security (carrier or affected shows clear by marker testing) or result in discarding a healthy dog (carrier or clear shows affected or carrier by marker testing.)
If only three chromosome types are available, the two verifying types plus one crossover, then if the marker gene is associated at times with the healthy allele, (mabHh) the result will include dogs which are affected or carriers by marker analysis which are genetically carriers or clears (false positives.) If the other chromosome type has the undesirable allele not always associated with the marker (maahd) the results will include dogs clear or carriers by marker analysis that are actually carriers or affected (false negatives.) However, the existance of one crossover chromosome type would make me suspicious that the other might also exist in the breed.
So are marker tests of any use at all?
Yes! In the first place, they demonstrate that the actual gene is on a relatively limited portion of a known chromosome. The marker gene can thus assist in finding and sequencing the gene actually causing the health problem.
In the second place, marker tests are accurate so long as neither parent of an individual has a crossover chromosome. In humans, such tests are most likely to be used when a problem runs in a particular family. The linkage of a marker with the genes actually producing the problem is generally based on studies of how the marker is linked to the genes in that particular family. With dogs, the verification is normally done on a breed basis, and the fact that breeds may actually be split into groups (color, size, country of origin) which interbreed rarely if ever is likely to be ignored. Dogs closely related via close common ancestors to the test population are the best candidates for marker testing. In general, keep up conventional testing side by side with the marker testing. If the marker testing and the conventional testing disagree (e.g, affected dog tests clear or clear dog tests affected) consider the possibility of a crossover, and notify the organization doing the test.
Basic Genetics IV:
the relationship of genes to traits (single locus)With the exception of the few DNA tests available, we cannot know the genetic makeup of our dogs, only the physical makeup, or phenotype. We tend to break that phenotype up into traits, some breed specific, some more general. For instance, we might know that a Sheltie is 15" tall, a black-nosed sable merle with full white collar, feet and Teletype and a narrow face blaze, OFA good, is missing one premolar, has natural ears, and had double rear decals. All of these "traits" are defined by human beings. Very few of them actually refer to single genes that might be inherited as dominant, recessive, incompletely dominant or co-dominant.
In some cases we can break down a trait into a specific combination of genes. In the case of color, for instance, we know of a considerable number of genes that affect color through specific processes. In some cases, this knowledge has fed back on what we consider to be traits. Thus in the case given, the dog is:
- Sable ay- (as opposed to black with or without tan-point markings).
- Black (as opposed to brown) B-
- Merle Mm
- Irish-marked sisi or sisw
- Possibly a face-marking gene
In addition, the dog's color can be affected by minor genes (such as the modifier genes determining how much of the dog is white) by random factors (which probably influence the exact pattern of both white spotting and the location of the dark patches in the merling) and by environmental factors (such as uterine environment, nutrition or excessive exposure to the sun.) The point is that very few of the traits that humans have chosen are in fact due solely to the effect of a single pair of alleles at a single locus. We have looked at some such simple traits as regards color.
However, the height of the dog, the ears, the hip rating, the missing premolar, and the double rear decals are probably not single-gene traits, but rely on the interaction of several pairs of genes, with perhaps some influence from the environment.
In general I am using dominant, recessive, co-dominant or intermediate to refer to genes at the same location on a single pair of chromosomes, i.e., alleles at the same locus. There are cases where genes at one locus can "hide" genes at another locus. An example in dogs is recessive yellow, ee, in which recessive yellow, although a recessive at its own locus, can hide whatever the dog carries at the A locus and the proposed K (dominant black) locus. This type of relationship among different loci is called epistatic. The locus that is hidden is referred to as hypostatic. In some cases (e.g., E at the E locus) an epistatic locus has an allele that allows the hypostatic locus to show its effects.
We will consider a number of types of inheritance. The first group actually refer to single-gene traits. Any of these types of inheritance may also be involved in the inheritance of multiple-gene traits.
Single-locus inheritance
More complex inheritance will be covered on the next page, and includes
- Modifier genes
- Polygenic additive
- Threshold traits
- Variable expression
- Incomplete penetrance
- Polygenic recessive or dominant
- Mixed polygenic
Dominant-recessive inheritance
Black and brown provide a clear example of a dominant-recessive relationship among alleles. Every dog has two genes at the black/brown locus. If both genes are for black, or if one is for black and one is for brown, the dog is black, most readily identified by nose color. If both genes are for brown, the dog is brown, again most readily identified by nose color. BB cannot be distinguished from Bb without genetic tests or breeding tests.
Many genetic diseases, especially those that can be traced to an inactive or wrongly active form of a particular protein, are inherited in a simple recessive fashion. van Willebrand's disease (vWD) for instance, is inherited as a simple recessive within the Shetland Sheepdog breed.
Intermediate inheritance
Warning! Although this type of inheritance is common, it has a variety of names (incomplete dominance and overdominance are two common ones) some of which are also used for other things entirely. Here I will use it to refer to the type of inheritance in which the animal carrying two identical alleles shows one phenotype, the animal carrying two different identical alleles shows a different phenotype, and the animal carrying one copy of each of the alleles shows a third phenotype, usually intermediate between the two extremes but clearly distinguishable from either.
In dogs, merle color is a good example of this type of inheritance. If we define M as merle and m as non-merle, we find we have three genotypes:
- mm non-merle, with normal intense color
- Mm merle, with normal color diluted in a rather patchy fashion
- MM homozygous merle, extreme dilution, dog mostly white if a white-spotting gene is also present, and often with anomalies in hearing, vision and/or fertility.
Note that there is really a continuum between dominant-recessive and intermediate inheritance. In Shetland Sheepdogs, for instance, sables carrying one gene for tan-point have on average more dark shading than dogs with two sable genes. However, the darkest shading on dogs pure for sable is probably darker than the lightest shading on dogs carrying a gene for tan-point. In practice, intermediate inheritance is often treated as if it were a special case of dominant-recessive inheritance, as can be seen by the symbols used for merle and non-merle - usually the capital letter refers to a dominant gene and the lower-case letter refers to a recessive gene. I think a separate name is justified because it could be equally well argued that homozygous merle is an undesirable recessive for which the merle color is a marker that the dog carries the merle gene.
Many of the standard color genes normally treated as dominant-recessive do in fact have intermediate inheritance, the heterozygote generally much more similar to one homozygote than the other, between at least some alleles in the series. Coat color gene loci with at least some allele pairs leaning toward intermediate inheritance include A (agouti, patterning of black and tan), C (color, intensity of color), and S (white spotting). I suspect the same is true for T (ticking), G (graying) and even D (dilution) if another diluting gene, such as merle, is present. This may be much more generally true than is recognized.
Co-dominant inheritance
The dividing line between intermediate inheritance and co-dominant inheritance is fuzzy. Co-dominance is more likely to be used when biochemistry is concerned, as in blood types. Co-dominance means that both alleles at a locus are expressed. Co-domininance in X-linked genes is a special case that will be treated under sex-linked inheritance.
Sex-limited autosomal inheritance
Please, don't confuse sex-limited inheritance with sex-linked inheritance. They are two totally different things. Sex-linked inheritance is discussed below. I do include sex-influenced traits under the sex-limited heading, though some genetics texts separate sex-influenced and sex-limited traits.
A classic example of a sex-limited trait in dogs is unilateral or bilateral cryptorchidism, in which one or both testicles cannot be found in their usual position in the scrotum. Since a bitch has no testicles, she cannot be a cryptorchid - but she can carry the gene(s) for cryptorchidism, and pass them to her sons. Likewise, genes affecting milk production are not normally expressed in a male. The main problem with sex-limited inheritance is that it is impossible to know even the phenotypes of the unaffected sex in a pedigree, which makes it difficult to determine the mode of inheritance.
In sex-influenced inheritance, the genes behave differently in the two sexes, probably because the sex hormones provide different cellular environments in males and females. A classic example in people is male early-onset pattern baldness. The gene for baldness behaves as a dominant in males but as a recessive in females. Heterozygous males are bald and will pass the gene to about 50% of their offspring of either sex. However, only the males will normally be bald unless the mother also carries the pattern baldness gene without showing it (female heterozygote.) If the mother is affected with baldness (homozygous) but the father is not, all of the sons will be affected and all of the daughters will be non-affected carriers. A bald man may get pattern baldness from either parent; a bald woman must have received the gene from both parents.
Sex-linked inheritance
In order to understand sex-linked traits, we must first understand the genetic determination of sex. Every mammal has a number of paired chromosomes, that are similar in appearance and line up with each other during gamete production (sperm and eggs). In addition, each mammal has two chromosomes that determine sex. These are generally called X and Y in mammals. Normal pairing of chromosomes during the production of gametes will put one or the other in each sperm or ovum.
In mammals, XY develops testicles which secrete male sex hormones and the fetus develops into a male. An XX fetus develops into a female. Thus sperm can be either X or Y; ova are always X. Sex linked inheritance involves genes located on either the X or the Y chromosome. Females can be homozygous or heterozygous for genes carried on the X chromosome; males can only be hemizygous.
X-linked recessive:
The most common type of sex-linked inheritance involves genes on the X chromosome which behave more or less as recessives. Females, having two X chromosomes, have a good chance of having the normal gene on one of the two. Males, however, have only one copy of the X chromosome - and the Y chromosome does not carry many of the same genes as the X, so there is no normal gene to counter the defective X.
An example of this type of inheritance is color blindness in human beings. Using lower case letters for affecteds, we have
- Affected male: xY Color blind
- Non-affected males XY Normal color vision
- Affected female xx Color blind
- Carrier female xX Normal color vision
- Clear female XX. Normal color vision
Now the possible matings:
xY to xx (both parents affected) xx females and xY males, all offspring affected.
xY to Xx (affected father, carrier mother) half the females will be xX and carriers, half will be xx and affected. Half the males will be XY and clear, half will be xY and affected.
xY to XX (affected father, clear mother) all male offspring XY clear, all daughters Xx carriers.
Note that the daughters of an affected male are obligate carriers or affected. The unaffected sons of an affected male cannot carry the problem.
XY to xx (father clear, mother affected) xY males (affected) and xX daughters (carriers.)
XY to Xx (father clear, mother carrier) half the males affected (xY) and half clear (XY); half females clear (XX) and half carriers (Xx)
XY to XX (father and mother both genetic clears) all offspring clear.
Note that all female offspring of affected males are obligate carriers (if not affected.) Likewise, any female who has an affected son is a carrier. Non-affected sons of affected fathers are genetically clear.
This type of inheritance may be complicated by the sublethal effect of some X-linked genes. Hemophilia A in many mammals (including dogs and people) is a severe bleeding disorder inherited just like the color-blindness above. Many affected individuals will die before breeding, but for those who are kept alive and bred for other outstanding traits, non-affected sons will not have or produce the disease. All daughters, however, will be carriers.
X-linked dominant:
Here I will use X+ for the dominant gene on the X chromosome, and X for the gene on the normal X chromosome. The actual possibilities are similar to those for an X-linked recessive, except that X+X females are now affected. In X-linked dominant inheritance, more females than males will show the trait. Possible matings are:
Affected to homozygous affected (X+Y to X+X+): All offspring affected.
Affected to heterozygous affected (X+Y to X+X): All daughters affected; half of sons affected.
Affected to homozygous normal (unaffected female): (X+Y to XX): All daughters affected, all sons normal.
Normal to homozygous affected (XY to X+X+): all offspring affected, but daughters are heterozygous affected.
Normal to heterozygous affected: (XY to X+X): Half of offspring affected, regardless of sex. Affected daughters are heterozygous.
Normal to normal (XY to XX) all offspring clear.
X-linked co-dominant:
Mammalian cells, even in females, get along fine with just one X chromosome. In fact, more than one X chromosome within a cell seems to be a problem if both are active. So in female cells, one or the other X chromosome must be inactivated. This occurs more or less at random, so any female mammal has patches of cells with one X chromosome inactivated, and patches with the other not active. If the gene being discussed codes for an enzyme that is spread throughout the body, it may not be obvious that the different patches of cells are behaving differently, and we will get what looks like dominant, recessive, or intermediate inheritance.
However, if the gene is expressed directly within the cell, the mosaic nature of the female may become obvious. The tortoiseshell cat provides an excellent example of this.
In cats, the orange color is on the X chromosome. It is designated as O, and the "wild-type" gene that allows black (eumelanin) to appear in the coat is designated +. Note that a cat homozygous or hemizygous (male) for + may be solid or tabby with the eumelanin pigment showing only in the tabby stripes, ticks and blotches (in extreme cases only on the tips of the hairs) and the "black" may just as well be chocolate or blue. A cat with only O genes will be some shade from cream to deep red., with no black/blue/chocolate pigment in the coat, but usually with tabby markings.
However, a cat with the gene for orange on one X chromosome and the gene for non-orange on the other is neither orange nor non-orange, but has patches of both colors. This color is known as tortoiseshell, and I am going to use the broad definition, including blue/cream or chocolate/yellow tortoiseshells. Most of the time cats with two X chromosomes are female, and since two X-chromosomes are required for tortoiseshell, most tortoiseshell cats are female.
Now and then a cell does not divide properly when it is making a germ cell, and you might, for instance, get an XY sperm cell. This would produce an XXY male, which would look male (he has a Y chromosome) but also have two versions of X and thus could be a tortoiseshell. However, the XXY makeup, corresponding to Klinefelter's syndrome in human beings, is believed to produce sterility. A similar syndrome involving females with only one X chromosome but no Y is called Turner's syndrome in human women, and again appears to produce sterility. We will therefore consider only matings between animals with two sex chromosomes.
Non-orange male to non-orange female (+ to ++): all non-orange offspring.
Non-orange male to tortoiseshell female (+ to +O): Males 50% orange and 50% non-orange; females 50% non-orange and 50% tortoiseshell.
Non-orange male to orange female (+ to OO): all males orange; all females tortoiseshell.
Orange male to non-orange female (O to ++): All males non-orange; all females tortoiseshell.
Orange male to tortoiseshell female (O to O+): males 50% orange and 50% non-orange; females 50% orange and 50% tortoiseshell.
Orange male to orange female (O to OO): All offspring orange.
Y-linked inheritance:
The Y chromosome in most species is very short with very few genes other than those that determine maleness. Y-linked inheritance would show sons the same as their fathers, with no effect from the mother or in daughters. In humans, hairy ears appear to be inherited through the Y chromosome. Padgett does not list any known problem in dogs as being Y-linked.
Canine Color Genetics Dogs have a wide variety of genes that influence color. Further, the same genes may give a very different effect on different types and lengths of coats. While this site is primarily concerned with Shetland Sheepdog colors and a long, working-type (double) coat, I will use comparisons from other breeds and even other species whenever it seems useful. References, including other mammalian color genetics, are on a separate page.
One of the biggest problems people have with genetics is the assumption that a defined trait - size, ear type, color, yappiness - is due to a single gene. In fact, genes code for two types of things. One, which is relatively well understood, is the structure of a particular protein. The normal equivalent of the albino gene, for instance, codes for tyrosinase, an enzyme which breaks up the amino acid tyrosine as a first step in producing melanin, the major pigment in mammalian skin and hair. In an albino, this enzyme cannot be produced, and as a result melanin cannot be produced. A second type of gene controls when and where other genes are turned on or off. These genes are the subject of vigorous ongoing study, and probably have a major impact on such things on the number of vertebrae in the spine or the age at which growth is complete. I've included a page which defines some of the terms used in genetics, as well as explaining dominant, recessive and incompletely dominant genes. Right now, let's look at some of the gene series (loci) known to influence canine color, and try to get a feel for what they do.
Before starting our list, we need to know that mammals have two forms of melanin in their coats. One, eumelanin, is dark, though it can vary somewhat in color due to variations in the protein that forms the framework of the pigment granule. The base form of melanin is black. Melanin can also appear brown (often called liver in dogs) or blue-gray. The second pigment, which varies from pale cream through shades of yellow, tan and red to mahogany (as in the Irish Setter), is called phaeomelanin. There are at least two and possibly as many as four gene series that determine where, on the dog and along the length of the hair, eumelanin and phaeomelanin appear.
The generally recognised color series (loci) in dogs are called A (agouti), B(brown), C (albino series), D (blue dilution) E (extension), G (graying), M(merle), R (roaning), S (white spotting) and T (ticking.) There may be more, unrecognised gene series, and in a given breed modifying factors may drastically affect the actual appearance. Thus one school of thought holds that the round spots on a Dalmation are due to the same gene that produces the roaned areas on a German Shorthair Pointer, but with vastly different modifiers.
A, the agouti series. The standard assumption, based on Little's research, is that this series contains four alleles (different forms of the gene). A fifth allele may exist in Shetland Sheepdogs, and a sixth in certain "saddle-tan" breeds.
- As produces black without any tan on the dog. White markings are due to a different gene, and there are other genes that can modify the black to liver (chocolate Lab) or blue dilute (blue Great Dane.) If As is present, in most cases the dog will be able to produce only eumelanin pigment (but see the E series). Note that the agouti series is known in a number of mammals, and dominant black is almost always found in a different series, so there is a strong possibility that dominant black is not really in the agouti series.
- ay in the absense of As produces a dog which is predominantly tan (phaeomelanin) sometimes with black tipped hairs or interspersed black hairs. The usual term for this color is "sable." In examining dogs from ay breeds, I have generally found that even if there is no other black on the coat, the whiskers (the course, stiff vibrissae, not the "beard" seen with some terrier coats) are black if they originate in a pigmented area. Examples of ay dogs include Collies, fawn Boxers and Great Danes, and some reds (Basenji red is thought to be ay, for instance.) ay is recessive to As, but incompletely dominant to at. That is, an ayat dog is on average darker (more black hairs) than an ayay dog, but the difference is generally within the range of color for ayay within the breed.
- at, present in double dose, produces a dog which is predominantly black, with tan markings on the muzzle, over the eyes, on the chest, legs, and under the tail. A Dobermann or Rottweiler is a good example of the classic black and tan pattern. The Bernese Mountain Dog shows the effect of black and tan combined with white markings, often called tricolor.
- aw is the fourth allele considered by Little. This is the wild "wolf-color" seen in Norwegian Elkhounds and possibly in some salt-and pepper breeds. It differs from sable in two ways. First, the tan is replaced by a pale cream to pale gray color. Second, the hairs are normally banded - not just the scattering of black-tipped hairs sometimes seen in a sable, but several bands of alternating light and black pigment along the length of the hair. Little was unable to determine the dominance relationship of this gene, or even to say with certainty that the banding and the reduction of tan pigment were due to the same gene.
Although Little did not make any distinction between the Dobermann black and tan and the "saddle tan" seen in many terrier breeds (black "saddle" but extensive tan on legs and head), it seems likely that a fifth gene exists in the a series. For the moment I'll call it "saddle tan," asa. It seems recessive to ay sable, but other dominance relationships in the series need more investigation.
Finally, at least two breeds (Shetland Sheepdog and German Shepherd) have a fully recessive black. Since black is the bottom recessive of the A series in many other mammals, it seems logical to assign this color to recessive black, a, and state that recessive black is caused by aa at the agouti locus. There is an alternative theory in Shelties which suggests the existence of a recessive gene that removes tan points from a genetic black and tan or a dominant, widespread gene that forms tan points on all colors but dominant black.
Little's assignment of dominant black in dogs to the A locus (As) is totally against experience with this locus in other species, where more yellow is generally dominant to more black. There may be a third locus controlling dominant black, in which case Ay would be the top dominant in the A series.
B, the brown series. This series is relatively simple. B, in single or double dose, allows the production of black pigment. A bb dog produces brown pigment wherever the dog would otherwise have produced black. The gene apparently codes for one of the proteins that makes up the eumelanin pigment granule, so the bb granules are smaller and rounder in shape as well as appearing a lighter color than those of a dog carrying B. This gene is responsible for a number of liver and chocolate colors, especially in the sporting breeds. The same gene produces some "reds" (in Australian Shepherds, Border Collies, and Dobermanns, for example), and probably the bronze Newfoundland. It has some effect on the iris of the eye and on the skin color, including the eye rims and the nose leather. Phaeomelanin (tan) is very little affected, so the color of the tan points on a red Dobermann (atatbb), for instance, is little affected. I have seen little discussion of the effect of brown on a sable dog, but I would expect a brown nose leather and eye rims, with the coat shaded brown rather than black. Probably the dog would closely resemble a sable, perhaps with an orangey cast and a light nose. Note that some shades of liver, though a eumelanin pigment, overlap some shades of tan, a phaeomelanin pigment. In particular the deadgrass color (bbcchcch) can overlap recessive yellow (ee)
C, the albino series. This again is a fairly complex locus, especially in other mammals. The top dominant, C, allows full color to develop, and is probably the structural gene for tyrosinase. The bottom recessive, c, does not appear to occur in dogs, but in other mammals it completely prevents the formation of any melenin in the coat or the irises of the eyes, giving a pink-eyed or red-eyed white. It is worth pointing out that human albinos from dark-skinned parents often show some yellowish or reddish hair and even skin color, but it seems this is not due to granular melenin. c, therefore, is a form of tyrosinase which cannot act as it is intended to in the formation of melanin. Since c is simply a non-working form, there may be more than one form of c gene (lots of ways to get something not to work), and there is some evidence that when two different forms are mated, colored offspring may result.
There are a number of intermediate genes where the mutation apparently produces a partly active form of tyrosinase. Some C alleles known in other mammals are:
- C full color, allows full expression of whatever pigment is prescribed by other genes. Most dogs are CC.
- cch, chinchilla or silver, when present in double dose removes most or all of the phaeomelanin pigment with only a slight effect on black pigment. This is named after a small fur-bearing South American rodent called the chinchilla. Black and silver replacing black and tan, or a wolf-like color without the extra banding (see aw, above) may also be due to a cchcch genotype. Dogs with very light tan probably are cchcch or something similar. Liver dogs show lightening even of eumelanin pigment, and the "deadgrass" color of the Chesapeake Bay Retriever is thought to be due to a bbcchcchgenetic makeup. The possibility of other, rufous modifiers affecting the shade of phaeomelanin pigment needs to be kept in mind, as does the possibility of more than one form of chinchilla in the dog - rabbits are thought to have three.
- ce, extreme dilution, has also been proposed for the dog. This gene may be part of the makeup of some "white" dog breeds where the white color is due to extreme dilution of tan. The West Highland White Terrier may be ceceee. A cross to a black and tan breed would be interesting from the point of view of color genetics. Eyes may be lightened in some species, but this is doubtful in dogs.
- ch, Himalyan, is not known to occur in the dog. In homozygous form, it makes the formation of eumelanin dependant on the temperature of the skin. Thus a genetically solid black animal will have reduced black on the extremities (seal brown) and an almost white color on the body. The effect on tan/orange pigment is confusing - the tan in agouti hairs is removed, but that resulting from the orange gene in cats (not in dogs) remains intense on the extremities. There is reason to suspect that this gene, as well as some forms of chinchilla, also affects the organization of the brain, particularly in the neural pathways from the eyes to the brain. There may be a reason for Siamese cats to be cross-eyed. Eyes are normally blue or pink.
- cp, platinum, is optically similar to albino but retains very slight tysonase activity and in the mouse is described as retaining some luster in the coat as opposed to the pure white seen in albino. Although there is a total absense of proof one way or the other, I would hypothesize that the white Doberman, with pale blue eyes and pink nose, is due to a homologous gene.
- c, albino, is known to occur in the dog RARELY , though possible candidates for mutations to c have been recorded. As mentioned above, the c gene cannot produce working tyrosinase, and a cc individual cannot produce melanin pigment.
As seen from the above, C is known to have a number of different forms and effects. The usual assumption is that dogs have at least one mutant allele, cchwhich when homozygous lightens phaeomelanin (yellow) pigment to cream and more weakly affects liver and longhaired black. A second proposed allele, ce may be responsible for further reduction of cream to white in some breeds, or modifying alleles may be responsible for the further lightening in these cases. While some forms of C modify eye pigment (e.g., blue eyes in Siamese cats) there is little evidence for this in dogs unless "white" Dobermans are indeed due to a C-locus mutation. Although C appears to be fully dominant over any of the other alleles, the dominance relationship between the others generally goes in the direction of more color incompletely dominant over less color, the heterozygote generally resembling but not necessarily identical to the homozygote with more pigment
D, the dilution series. This, again, is a relatively simple series, containing D (dominant, full pigmentation) and d (recessive, dilute pigment). In contrast to C, which has its strongest effect on phaeomelanin, or B, which effects only eumelanin, D affects both eumelanin and phaeomelanin pigment. It is thought to act by causing the clumping of pigment granules in the hair. Like B, it often affects skin and eye color, and in some breeds dd has been associated with skin problems. "Maltese blue" is a term often used to describe dd blacks. If a solid liver dog also is dd, the result is the silvery color seen in Weimararners and known as "fawn" in Dobermans. (In most breeds, fawn refers to ay yellows.)
While dd acting on black or liver is a part of the genotype of several breeds, dd acting on sable is relatively rare. For one thing, the action of dd on phaeomelanin has been described as a flattening or dulling of color. The cinnamon color in Chows is probably due to an ayaydd genotype, but otherwise the combination of dd with phaeomelanin coat color seems limited to breeds in which color is of little importance (e.g., blue brindle in Whippets.)
Although D is usually described as completely dominant to d, I have seen one blue merle Sheltie bitch who suggested that this may not always be the case. The black merling patches in this bitch were actually an extremely dark blue-gray. Other than this she was an excellently colored blue merle. The owner insisted that she was not a maltese blue, but that she had relatives who were. I suspect that this bitch may have been Dd, with the additional diluting effect of the merle gene allowing the normally hidden effect of a single dose of d to show through.
E, the extension series. This series is probably the least satisfactory of those generally assumed to exist in the dog. In most mammals, the E series includes Ed (dominant black), E (normal extension) and e (recessive red or yellow, and sometimes some intermediate alleles called Japanese brindles. In dogs, this is clearly not the case; breeding experiments have conclusively proven that dominant black and recessive red are not in the same series. This has led to dominant black being thrust into the A series, which as already mentioned conflicts with results in other mammals.
In this summary, I will give the genes as postulated by Little, followed by a brief discussion of other possible explanations and a suggestion for matings that might clarify the situation. Note that the question is not in whether the genes occur, but whether they are in fact alleles in the same gene series. With regard to e and E, recent sequencing of the e and E genes in dogs show definite homology with those in other species.
- Em, mask factor. This gene replaces phaeomelanin (tan) with eumelanin (black) over part of the dog. There is considerable variation in the area of replacement, probably affected by modifiers but possibly involving more than one form of Em. At its weakest the mask factor may produce black hair fringing the mouth, or a slightly smutty muzzle. At its strongest (Belgian Tervuren) most of the head is black, and there is considerable blackening of chest and legs. The effect of Emshows to its fullest extent on clear sable dogs (ayay), but is visible on the tan points of black and tan dogs (atat) as well. In its strongest version, it can change a black and tan to a pseudo-black, with tan so restricted in its distribution that it may not be immediately apparent that the dog is not black. The occasional "black" puppy produced by two Tervuren parents is probably this type of black, with two ayatEmEm parents producing an atatEmEm puppy. A similar but not quite as strong blackening of the head of a genetic black and tan occurs in German Shepherds.
- Ebr, brindle. This gene probably got into the E series by mistaken homology with Japanese brindle, which behaves quite differently from brindle in the dog. In Japanese brindle, the patchy color is believed to be due to two alleles of the E series side by side on the same chromosome. Only one can be expressed, and different parts of the animal will show the expression of different genes. The result is a coat made up of random small patches of tan and black pigment, rather like a tortoiseshell cat. If a Japanese brindle animal also has the genes for extensive white spotting, the tan and black pigmented areas tend to become larger and more compact, similar to what one sees in a calico cat (genetically, a tortoiseshell with white markings.) There is a canid which might be Japanese brindle with white spotting, the Cape hunting dog,Lycaon pictus. This animal has a coat which is a rather random patchwork of black, yellow and white. The color has very little similarity to brindle in the dog.
Brindle in dogs consists of black, vertical stripes on a sable/fawn background, usually rather soft-edged, but much more regular that a typical Japanese brindle, and showing no tendency for the tan and black patches to become more distinct in the presense of white spotting genes. Genes that affect eumelanin will affect the dark stripes, so a bb brindle, for instance, will have brown rather than black stripes. Brindle on a black and tan will show only in the tan areas, while brindle on a black cannot be distinguished at all. If in fact recessive red (ee) is in the same series with brindle, it is not possible for brindle (or mask) to occur on an ee dog as one of the E genes would have to be Ebr (or Em), leaving no room for ee. Little implies that brindle and mask were co-dominant, with masked brindles being EbrEm, in which case masked brindle could not breed true.- E, normal extension of black, allows the A-series alleles to show through with no masking or brindling. It is apparently recessive to both Em and Ebr.
- e, recessive red, overrides whatever gene is present at the A locus to produce a dog which shows only phaeomelanin pigment in the coat. Skin and eye color show apparently normal eumelanin, although some ee dogs appear to show reduced pigment on the nose, especially in winter (snow nose.) A number of breeds show recessive red as a normal or even breed-wide characteristic - Irish Setters, Golden Retrievers, yellow Labradors. In a few breeds such as the Cocker Spaniel "reds" may be either ayay or ee, and crossing the two can produce unexpected blacks. I believe there may be a key in the color of the whiskers, which on my observations seem to be black in ayay breeds and straw to cream (dilute red) in ee breeds, always assuming the whisker base sprouts from a pigmented area. Little hypothesized that dogs with both forms of red (ay-ee) were not viable and would be lost before birth.
The dominance relationships in the Little proposal are not simple. He assumes that Em and Ebr are co-dominant. In an ayay dog, then, brindle without a mask could be EbrEbr, EbrE, or Ebre. A masked dog without brindling would be EmEm, EmE or Eme. A masked brindle would have to have the genotype EmEbr. This assumption makes some predictions which should be readily testable:
- Two masked brindles, mated together, should produce appoximately a 1:2:1 ratio of masked fawn to masked brindle to brindle without masking. In other words, masked brindle should not breed true.
- A masked brindle could not carry E or e. Thus a masked brindle, bred to sable ayayE- would pass either mask or brindle. The expectation would be a litter of brindles without masks and masked sables (fawns) without brindling, but no sables without either mask or brindle and no masked brindles.
- If a masked brindle is bred to an ee red, the results would depend on the A series genes in the ee red, but there would be neither ee nor ayay reds with neither masking nor brindling. Some blacks might occur, but if the puppy had areas of tan pigment, the tan would be either masked or brindled, but never both and never tan without either mask or brindle.
My impression in talking to breeders of masked brindles is that these predictions are not fulfilled. Possible revisions of the E series include:
- Remove Ebr from the E series, instead recognising that in many ways it is closer to tabby (Ta) in the cat family. This is the gene series responsible for the various stripes, ticking, spots and rosettes seen in both wild and domestic cats. Granted, the pattern is not the same (striped cats normally have stripes ringing the legs), but brindle is also a black striping gene which is visible primarily on an ay background. This would leave Em, E and e in the E series, giving a prediction that Em- bred to ee could produce either 100% masks if the mask is EmEm, half masks and half sables without masks if the mask is EmE, or half masks and half recessive reds if the mask is Eme. The one outcome that would be missing is that a masked to recessive red breeding could produce unmasked sables and unmasked recessive reds in the same litter. Given the difficulty in distinguishing sables from recessive reds, this might prove difficult.
- Remove Ebr from the E series, possibly putting it in the same series with dominant black (currently in the A series.) The new series (here called K - the last letter of black - for convenience) would have three genes, Kddominant black, Kbr producing eumelanin stripes on any phaeomelanin (tan) pigment on the dog. The assumption is that Kd is dominant over Kbr which in turn is dominant over k (more black dominant over less black.) The prediction would be that a dominant black (Kd-) bred to a clear sable would produce either all dominant blacks if the black is KdKd, a fifty fifty mix of dominant black and brindle if the black is KdKbr, or a fifty fifty mix of dominant black and clear unmasked sable if the black is Kdk, but never a litter with all three colors. Unpublished studies on racing greyhound litters agree with this prediction.
- Em might still be in the E series, but this should be tested. The test breeding would be difficult, because of the difficulty in being sure whether a "red" dog is ee or ayay, but the test is whether a masked dog, bred to another mask or to a recessive red ee, produces both ee red and fully expressed, unmasked tan-point or sable in the same litter. Probably some cross breeding would be required to be sure of the genotypes of parents and offspring.
- If both removals hold up, this would leave the E series with just two alleles, normal expression of the A series (E -dominant) and recessive red (e - recessive.) It has now been reported in the scientific literature (Newton et al, 2000) that the genetic sequence of canine e/E correponds to the E-locus (specifically recessive red) in several other species (fox, cow, human and mouse.)
G, the graying series. Although only two genes were recognised in this series by Little, this may be a more complex locus, or genes that affect graying may reside at more than one locus. The effect of G, in single or double dose, is the replacement of colored by uncolored hairs as the animal ages, very much like premature graying in human beings. This gene should be suspected in any breed where a dark puppy pales and washes out with age, and the paling is due to interspersed white hairs. The gene is almost certainly present in some Poodles, Old English Sheepdogs, and terriers. The fading may start immediately after birth or after a period of weeks to months has elapsed, and may go as far as it is going to by the first adult coat or may continue through the animal's lifetime. G may or may not be the gene involved in the graying of muzzle and over the eyes in aged dogs, or in the lightening of black to steel blue without interspersed white hairs. This is a series that definitely needs more work.
M, merle. This is another dilution gene, but instead of diluting the whole coat it causes a patchy dilution, with a black coat becoming gray patched with black. Liver becomes dilute red patched with liver, while sable merles can be distinguished from sables with varying amounts of difficulty. The merling is reportedly clearly visible at birth, but may fade to little more than a possible slight mottling of ear tips as an adult. Merling on the tan points of a merled black and tan is not immediately obvious, either, though it does show if mask factor is present, and may be discernable under a microscope. Eyes of an Mm dog are sometimes blue or merled (brown and blue segments in the eye.)
Although merle is generally treated as a dominant gene, it is in fact an incomplete dominant or a gene with intermediate expression. An mm dog is normal color (no merling). A Mm dog is merled. But an MM dog has much more white than is normal for the breed (almost all white in Shelties) and may have hearing loss, vision problems including small or missing eyes, and possible infertility (Little). The health effects seem worse if a gene for white markings is also present. Thus the dachsund, which is normally lacking white markings, has dapples (Mm) and double dapples (MM) the latter often having considerable white, but according to Little other effects are limited to smaller than normal eyes. In Shelties, Collies, Border Collies, and Australian Shepherds, all of which normally have fairly extensive white markings, the MM white has a strong probability of being deaf or blind. The same is probably true with double merle Foxhounds and double merles from Harlequin Great Danes with the desired white chest. A few double merles of good quality have been kept and bred from, as a MM double merle to mm black breeding is the only one that will produce 100% merles.
It is possible that merle is a "fragile" gene, with M having a relatively high probability of mutating back to m. The observed pattern would then be the result of some clones of melanocytes having suffered such a back mutaion to mm while they are migrating to their final site in the skin, producing the black patches, while others remained Mm. This hypothesis also explains why a double merle to black breeding occasionally produces a black puppy, the proposed back mutation in this case occurring in a germ cell. On the other hand, the observed blacks from this ype of breeding may actually be cryptic merles - genetically Mm, but with the random black patches covering virtually all of the coat.
Merle is a part of the pattern of ragged black spots seen in the harlequin Great Dane. There appears to be an additional gene which removes the dilute pigment, leaving the "blue" area clear white. The fact that harlequins continue to produce merles argues that animals pure for this proposed extra factor may not exist, and one possibility is that a homozygote for this whitening factor is an embryonic lethal. Interestingly, there are recent reports of Shelties born with a harlequin pattern, but in this case the "blue" area actually develops color with time, winding up a light silvery blue. These dogs appear to have larger than normal black areas, at the extreme being so-called cryptic merles, that is, no blue is visible without an extensive search. Other shelties born harlequin or "domino" retain the white body color.
Although Danes are usually solid color, the harlequin color description includes a preference for a white neck and front. Since the black patching is as apt to be on neck and front as anywhere else, this requires incorporation of a gene for white spotting (probably irish spotting, sisi). Given that SS double merles seem to fare better than their si sicounterparts, I would expect that double merles from harlequin Danes with patched fronts and necks might be healthier than from those that fit the standard better. The harlequin description also faults black hairs in the white area. The harlequin - silver blue pattern in Shelties could be an extreme case of black hairs in the white area. Both harlequins and the silver-blue merle Shelties have occasional patches of gray (merle?) as well as black, though this is not considered desirable.
R, roan. This may or may not be a true series. Both Little and Searle suggest that roan may simply be a very fine ticking, with dark hairs growing in an initially white area of the coat. A second type of roan, in which white hairs develop in an initially dark coat, could be due to gray or could be a type of roaning different from the progressive development of dark hair in a light area. In any event, roan (R) appears to be dominant to non-roan (rr). It is not clear whether this is full dominance or incomplete dominance. I will here treat roan as being at the ticking locus.
S, white spotting. This is another somewhat unsatisfactory series, and one in which modifying genes appear to have a very large effect. Certainly there are genes for solid color, for a more regular white spotting, and for basically white with some colored markings. But the variability within each type makes it unclear how many alleles actually occur at this locus. In general dominance is incomplete, with more color being dominant over less color. Heterozygotes commonly resemble the more-pigmented homozygote, but with somewhat more white.
- S, solid color. This is the normal gene in breeds without white markings. An SS dog can completely lack white, but it can also express very minor white markings - white toes, white tail tip, or a star or streak on the chest. SS breeds generally fault these markings.
- si, irish spotting. Irish spotting is generally confined to the neck, the chest, the underbody, the legs and the tail tip. White does not cross the back between the withers and the tail, though it may appear on the back of the neck. Breeds with "Collie markings" which breed true for the markings are generally si si.
- sp, piebald. This is a more difficult gene to identify. Certainly some breeds, such as parti-color Cockers, seem to breed true for piebald. Crosses of parti-color and solid in Cockers, however, often have minor white marking. Piebald and irish spotting seem to overlap in phenotype in one direction, while piebald and extreme white overlap in the other. In general, it seems a piebald has more than 50% white, white often crosses the back, and the pattern gives the impression of fairly large colored spots on a white ground.
- sw, extreme white piebald. Extreme white piebalds range from the color-headed whites (Collies, Shelties) which may also have a few colored spots on the body, especially near the tail, through dogs with color confined to the area around the ear or eye (Sealyham, White Bull Terrier, Great Pynenees) to some pure whites (Dalmation ideal). There is some anecdotal evidence that swsw dogs without color on or near the ear have a higher probability of deafness than dogs with color on the ears, but this varies with breed and it is not known whether a separate allele of S might be involved. In Boxers, some whites are produced from show-marked parents. Little believed that the Boxer lacked the gene for si, the irish-type spotting desired in the show ring being produced by heterozygosity for S and sw. Since the Boxer club is adamantly opposed to any breeding of whites, even test breeding, this has not been independantly confirmed.
All of the spotting genes are assumed to be affected by the action of modifiers, with + (plus) modifiers being generally understood to increase the amount of pigment (decrease white) while - (minus) modifiers being assumed to decrease the amount of pigment (increase white.) Merle appears to act as a minus modifier, in addition to its effects on coat color.
It is not clear to what extent the S series affects head pigment. Color-headed white shelties, for instance (swsw), can have completely colored heads - not even a forehead star or white nose. On the other hand, relatively conservatively marked dogs can appear with half white or all white heads. There is probably at least one other gene series that affects head markings. It is at least possible that the plus and minus modifiers affect head and body markings simultaneously.
T, ticking. Some dogs develop flecks of color in areas left white by genes in the S series. The clearest and most obvious ticking is seen in Dalmations, where additional modifier genes have enlarged and rounded the ticks. A large number of irish, piebald and extreme white breeds also have variable ticking, though not often as obvious as the Dalmation. The color of the ticking seems to be the color the coat would be in that area if the white spotting genes were not present. Thus a genetically black and tan Dalmation (a fault) will have tan spots where a black and tan would have tan markings. A ticked sable, ayayTT or ayayTt, may not have obvious ticking, becasue there is not much contrast between the tan and the white. Careful examination, however, will often show tan flecks on the legs. Ticking on a long-haired dog is also difficult to discern. The Border Collie on the front page of my site is ticked and probably sisw, as well as having the gene(?) for half white head. The tick marks in her ruff are not visible in the photo, but they are present (if difficult to find) on the living dog.
The usual dominance relationship given is that T (ticking) is dominant over t (lack of ticking.) Some breed-specific sources suggest that ticking acts as a recessive. I am inclined to suspect incomplete dominance of T. In Border Collies, for instance, a color called blue mottle is in fact a very heavily ticked piebald. The dam of the Border Collie mentioned above was such a blue mottle, presumably TT, while Dot is apparently Tt.
Ticking is also very much affected by genes which modify the size, shape and density of tick marks. In fact roan, which can develop by the gradual growth of pigmented hair in white areas of the coat, may simply be a form of ticking.
Size Genetics
The purpose of the following article is to give an explanation about how breeders are able to breed a dog for a certain size. This article uses the Sheltie breed as an example. This theory is also true for the APBT.
Size as an example of additive inheritance Sheltie breeders, as a group, tend to be hung up onsize. We have reason to be. The breed was produced by mixing large and small breeds, few if any of which were in the size range we accept as correct today (13" to 16", with preference for 15" and up). Size, however, is not a simple genetic trait. At a minimum, it depends on the genetic codes for growth hormones, the genes that dictate when and how much growth hormone is produced, probably genes that code for receptor proteins that respond to growth hormones, genes that control bone shape and angulation between bones, and other genes affecting various metabolic processes. We don't even know the whole list, or how to determine what makes a particular dog large or small. In some cases the gene for larger size would be dominant, in some cases recessive, in some cases the dog heterozygous at a particular locus would be intermediate is size. It is, however, possible to use a very simple model to explain some of the oddities of Sheltie size. Remember this is a greatly simplified model! The real situation is almost certainly more complicated. We may even have a few genes for correct size hidden in there somewhere!
Suppose we assume we have four loci affecting size. Assume also that each locus has two alleles, one derived from the Collie part of our breed's ancestry, and the other from the original small island Sheltie (remember that at one time 12" was pushed as the maximum height) and toy breeds crossed in late in the 19th Century. We'll call these genes f, i, j, and k. The alleles for large size will be f+, i+, j+ and k+; those for small size will be f-,i-, j-, and k-. The size of the dog will be a base of 15" plus 3/4 times the sum of the "+" genes minus 3/4 times the sum of the "-" genes. A dog with all "+" genes, for instance, would be 21" tall, while a dog with all "-" genes would by 9" tall.
Suppose a breeder, breeding fairly close within her own line and related dogs, winds up with a consistent size genotype of f+f+i+i+ j-j- k-k-. All gametes will be f+i+j-k-. All puppies will have the same size genotype as their parents, and will be 15" tall. But she's had to inbreed quite a bit, and she is looking for an outcross that will give her back what she's lost without sacrificing her predictable size.
She finds another breeder, again breeding fairly closely within her own line, who also gets all 15" Shelties, and whose line is strong for exactly the traits she needs. Breeder A breeds her best bitch to breeder B's best stud dog, and breeder B, who is missing a couple of things A has managed to fix, breeds her own bitch to a stud from breeder A's lines. The puppies arrive, grow up, and all are 15".
Then two of these 15" pups, from litters level in size stemming from strains level in size, are bred to each other. The result could easily be puppies all over the map in size. What happened?
Breeder B had a consistent size genotype of f-f-i-i- j+j+ k+k+, and her dogs consistently produced f-i-j+k+gametes. The uniformly in -size puppies from the strain cross, then, all had the genotype f+f-i+i-j+j-k+k- and could produce any of 16 types of gamete, ranging from f+i+j+k+ to f-i-j-k-. I'm not going to try to draw a 16 x 16 Punnett square, but the expected size distribution in 256 puppies is:
1- 9 inch (all -)
8- 10 1/2 inch (7 -, 1 +)
28- 12 inch (6-, 2+)
56- 13 1/2" (5-, 3+)
70- 15" (4+, 4-)
56- 16 1/2" (5+, 3-)
28- 18" (6+, 2-)
8- 19 1/2" (7+. 1-)
1- 21" (all +)If we assume some minor size genes as well, so the various categories are smeared out somewhat, the results don't look too unfamiliar. Note that if you breed the 16 1/2" but do not breed the 13 1/2", the result will be a gradual loss of - genes, and an overall upward creep in height. Also, there is no way to look at a 15" dog and determine whether it is fully heterozygous ( f+f-i+i-j+j-k+k-) or homozygous ( f-f-i-i- j+j+ k+k+, for instance) It's not as simple as breeding only from in-size dogs with in-size littermates!
How about genes for correct size? Could we have an additional allele, f, i, j, k at each locus, with ffiijjkk dogs being uniformly 15", and dogs with 7 normal genes and one + gene being 15 3/4"? It would certainly be nice, as then we'd just have to eliminate the + and - genes to have a breed that breeds true for size. It would be a slow process, if only because dogs with the alleles for correct size would so easily be confused with dogs with a balance of + and - genes. Given the background of our breed, though, the source of such genes for correct size is an open question. Most of our breed's ancestors were larger or smaller than 13" to 16". The standard advice on breeding for size, though, is to breed to correct size, which is based on the unstated assumption that the alleles f, i j and k exist.
Other complications undoubtedly occur. The hypothetical small and large genes may differ in their effect - f+might contribute more to oversize than j- does to undersize, for instance. There may be additional loci that have a dominant-recessive effect - NN or Nn might add 2" to the height while nn would allow the fjkl loci to control size. On the other hand, QQ or Qq might allow the fjkl loci to control size while qq would be 2" less than the fjkl size. The important thing to remember is that size is based on more than one gene pair, and as a result can do some very strange things.
DNA specific tests can now be carried out for the presence of most of the colour alleles, particularly where one wants to know if there are unwanted dilution factors hiding within individuals. While it can look very complicated, try to understand the subject and thus produce the colours you want from matings and not waste litters with incorrect colours. Knowledge of your proposed breeding pairs’ colour genetics can help maximise desired colour combinations.
General information
Melanocytes are the cells that produce skin and hair colour and they are derived from neural crest cells. These cells arise along the back very early in foetal development and then give rise to a number of cell types, including a large proportion of the peripheral nervous system. If there is a decrease in the number of neural crest cells, other cell types are favoured, leading to a reduction in melanocyte formation (see below). The melanoblasts (immature colour cells) migrate from the dorsal midline over the surface of the body, so the last areas to be reached are the feet, chest and muzzle (ie where you are more likely to see white toes, etc) As you would see in a "Mis-mark " Pomeranian for example.
Neural crest cells also form part of the nervous system for the inner ear and eye. Animals selected for extreme white spotting (eg. Dalmatians) can have hearing and/or vision problems in other extreme white patterns (merle series). Dalmatian deafness is thought to be as a result of the absence of melanocytes in the stria vascularis of the inner ear.
Melanoctyes produce melanin by the action of alpha MSH. Large amounts of MSH results in Eumelanin (mainly Tyrosine) which produces black or derivatives of black (blue, chocolate, brown, liver). Restricted amounts of MSH result in Phaeomelanin (contains varying amounts of cystine and tyrosine) which produces reddish brown or yellowish tan.
#Control of melanoctye function is intricate and many loci(genes) have mutations which affect components of melanogenic control mechanisms.
There are about 10 recognised "loci" of different colour genes. Each locus can have a variable number of alleles that can influence the colour outcome (dilution, pattern, dominant or recessive effects).
All dogs carry these genes, many of which are in a fixed (homozygous) form eg. Gordon Setters, Elkhounds etc. In these breeds virtually all loci are fixed and there is very little colour variation across the breed. In other breeds some loci are fixed, while others have a degree of variation (number of alleles) present at other loci. Not all breeds carry all the possible alleles at each locus.
Current research - Many of the colour loci are being extensively studied to precisely locate the position of the various genes on the correct chromosome, and further, which alleles actually occur at that site and how they affect the colour outcomes available. From this, DNA markers may be developed, and thus allow DNA colour testing in various breeds prior to mating. The DNA research that is being done at this time is going to continue to change our understanding of colour genetics - its a fairly dynamic field - it is certainly not fixed in concrete.
Gene / allele designation - The way I write the alleles is to put the loci first as a capital. If only 2 alleles occur (dominant and recessive) then eg. D means the dominant allele, d the recessive allele. Where there are more than 2 alleles for a loci, I find it easier to keep the capital as the indicator for the loci, followed by a smaller letter indicating the allele. (If one has a fancy typewriter/computer, one could do the allele in smaller letters above the series loci letter.) If there are several alleles at a loci, these are usually written in decreasing order of dominance (according to current knowledge).DID YOU KNOW......................a Pomeranian puppy can be born almost black and end up a very light creme color three years later as an adult ? It is fascinating to see the changes in Pomeranian coat color as they grow from birth to adulthood . Some great examples are documented superbly by
There are several coat colors that can be associated with health concerns.
http://skyway.usask.ca/~schmutz/conditions.html
One such gene is MLPH or the D locus. Several, but not all dogs with a d/d genotype have Color Dilution Alopecia and/or Black Hair Follicular Dysplasia. Although d/d dogs could be blue, grey, pale brown or a dilute red, it is unlikely that the orange or cream of Pomeranians is due to this genotype since blue or grey are not listed as allowed colors and I have not seen photos of such dogs. In addition, there are black-and-cream Pomeranians which have a decrease in phaeomelanin on their undersides to cream but no decrease on their backs which remain black. It is therefore likely that it is the I gene that causes the variation in shade in orange (e/e) dogs.
Another gene that causes major health concerns is AP3 which causes Grey Collie Syndrome. Luckily this has only been identified in Collies since it is lethal to pups.
Yet another gene in the "blue" family that can cause health issues is merle. Merle can not be seen in dogs with an e/e genotype. This e/e genotype occurs commonly in Pomeranians since orange, red and white probably account for the majority of Poms. The problem is that M/M (homozygous merle) dogs are always deaf based on our studies. We recently genotyped 24 mostly white Australian Shepherds and all tested M/M (based on the Clark et al. 2006 PNAS published test) and all were deaf. A proportion of these dogs were also blind in one or both eyes since microphthalmia is another common side effect in M/M dogs. Although in many breeds it is possible to educate breeders to never breed two merle dogs together this advice is not possible to follow in Pomeranians since e/e dogs would not show the merle pattern. It would therefore be necessary instead to advise all persons who breed a merle dog to use only a black or sable mate or to have DNA testing done on their red, orange or white mate prior to breeding to be sure it did not carry merle.

This is a photo of a homozygous merle puppy with microopthalmia (small eyes). Note how small the eyes are compared to those of a normal puppy. One or both eyes can show reduction in size, and one or both eyes can be missing completely (anopthalmia).
To determine whether or not a Pomeranian carries the merle gene, a DNA test is now available. This test is only available through GenMark at a cost of $95.00 - see http://www.genmarkag.com/home_companion.php. Because the merle gene can be carried by an apparently normal dog, those that want to avoid this cruel gene will now have to add the expense of merle DNA testing to the other health tests that they already perform. Even those that want to produce merle puppies will have to perform this test to ensure that they are not breeding two merle gene carriers to one another since you cannot always tell by a dog's appearance if it has merle in its genetics
Although albinism is rare in dogs, it does occur. The side effects associated with albinism have not been well documented since the phenotype is so rare and may be caused by different genes in different breeds, each with different side effects. This should not be confused with white dogs that have deeply pigmented nose leather and pads however which do not have health concerns. The occasional white dog with pigmented leather is M/M but usually dogs of this genotype has splashes of mottled color on their body.
ALBINO
Sheila Schmutz, Ph.D.
Professor, University of Saskatchewan
Prior to 1996 the AKC standard read: red, orange, cream, sable, white, parti-color, black and black-and-tan. The main advantages of specifying coat colors are:
provide educational information to owners and breeders
provide information to judges
provide information to geneticists who are then able to tailor a DNA test package which identifies the alleles for these specific colors
identify which colors are excluded for either health, aesthetic or historical reasons
I would advocate this approach to most breeds. Pre-1996 the major genes and alleles causing variation in Pomeranian coat color were likely:
A (agouti) = agouti signalling protein (ASIP)
ay fawn (cream to yellow to red with darker tips)
sable (some solid black hairs intermingled amongst reddish hairs)
at black-and-tan or brown-and-tan
E (extension) = melanocortin receptor 1 (MC1R)
EM melanistic mask
E eumelanin can be produced
e only phaeomelanin produced
K (from blacK......"dominant black") (gene identified but not published yet)
K solid black, brown or blue (eumelanin pigmentation only)
k expression of agouti alleles that express phaeomelanin possible
I (dilues only phaeomelanin) = ? gene (Co-Dominant)
I intense, not diluted
i co-dominant decrease in intensity
S (spotting) = ? gene (in pigs this is KIT but may not be just one gene in dogs)
S solid colored
si Irish spotting
sp piebald or random spotting
Sheila Schmutz, Ph.D.
Professor, University of Saskatchewan
DID YOU KNOW THAT YOU CAN DO Color Testing FOR - A Locus ? YOU CAN FIND OUT IF YOU'R POMERANIAN IS REALLY A WOLF SABLE | |||||||||||||
ASIP, or agouti signalling peptide, is a gene affects pigmentation of coat color in dogs. This protein interacts with the melanocytes to stimulate production of melanin. This gene exists in many forms, or alleles, in dogs. The first of these alleles is generally known as AW, or the wild-type allele. This appears as a "wolf-grey" type of pattern. There is no direct test for this allele, and it is thought that this is the most dominant allele. The second allele, known as AY, is a dominant allele responsible for fawn or sable pigment. A dog only needs 1 copy of the AY allele to be either fawn or sable, depending on the breed. This allele is responsible for the coat color seen in dogs such as Pugs or French Bulldogs. In terms of dominance, it is thought that AW is the most dominant, followed by AY. A third allele is the "tan points" allele, or at. There is no direct test available for this allele at this time, however, it is expressed in dogs that do not carry the AY allele. This phenotype is common in dogs such as Doberman Pinschers or Geman Shepherds. The fourth allele is known as "recessive black," or the a-allele. This variant of the agouti gene causes a dog that does not carry the dominant black gene to be solid black. The recessive black allele is responsible for all-black German Shepherds, for example. The recessive black allele is recessive to all other alleles, meaning that the dog must carry two copies of the "a" allele to express this pattern. The Agouti gene is only visible in dogs that do not carry the dominant black gene. The dog can still carry any of the agouti alleles, however, this effect is hidden by the dominant black gene.
Cost
AY-Allele Results:
a-Allele Results:
|
WANT TO KNOW IF YOU REALLY HAVE A BLUE POMERANIAN | |||||||
The MLPH gene codes for a protein called melanophilin, which is responsible for transporting and fixing melanin-containing cells. A mutation in this gene leads to improper distribution of these cells, causing a dilute coat color. This mutation is recessive, so two copies of the mutated gene, or "d" allele, are needed to produce the dilute coat color. This mutation affects both eumelanin and phaeomelanin pigments, so black, brown, and yellow dogs are all affected by the dilution. A dilute black dog is generally known as "blue," though other names do vary for different breeds, such as charcoal or grey.
Cost
D-Allele Results:
|
Because TYRP1 is only associated with eumelanin, this mutation only has an effect on dogs that are "EE" or "Ee" at the E-locus. Dogs that are "ee" only produce phaeomelanin in their coats, so a mutation at the B-locus will not have an effect on their coat color. However, eumelanin is still produced in the foot pads and noses of "ee" dogs, so the B-locus still has an effect on these areas. Dogs that are "eebb" will have a brown nose and foot pads, rather than black.
B Locus Testing
Animal Genetics currently offers a test for the B-Locus to determine how many copies of the recessive "b" allele a dog carries.
Cost
$40.00 US for the B-allele test.
Sample Collection
Collect sample using buccal swabs provided by Animal Genetics. Ensure that the dog has not eaten within a few hours of sample collection. Any food particles can inhibit the test. Rub each of the swabs along the inside of the dog's mouth for 10-15 seconds, and allow the swabs to dry thoroughly. Label the provided envelope with the dog's name, and place the swab inside it. Download and complete a submission form for each sample and send along with payment to Animal Genetics for testing.
WANT TO KNOW IF YOU REALLY HAVE A CHOCOLATE OR BEAVER POMERANIAN ? |
TYRP1, or tyrosinase-related protein 1, is a protein that plays a role in the synthesis of the pigment eumelanin. In the dominant form of this gene, or the "B" allele, normal eumelanin is produced in the coat, and thedog's coat appears black in color. A mutation in the TYRP1 gene can occur causing a change in function which dilutes the black color pigment to a brown color. This mutated gene is known as the "b" allele. When a dog is homozygous for the mutation, meaning he has 2 copies of the recessive allele, the dog's coat color will be brown in color. This color can also be referred to as liver, chocolate |
Results are given using the following symbolic notation:
B/B | The dog carries two copies of the dominant B allele. The dog will have a black-based coat, and will always pass on the "B" allele to any potential offspring. All offspring will also be black-based dogs. |
B/b | Both the dominant and recessive copies of the B allele are present. The dog will have black-based coat, but carries the allele responsible for the brown phenotype. The dog can pass on either allele to potential offspring. |
b/b | Two copies of the recessive allele are present. The dog has a brown-based coat, as well as a brown nose and foot pads, and will always pass on the recessive allele to all potential offspring. |
Coat coloration is controlled by several different genes in dogs. One of these gene is referred to as "Dominant Black." The presence or absence of this gene determines if a dog can express "agouti" color patterns.
The Dominant Black gene consists of three different alleles, or variants. The first allele, which is dominant, is noted as "KB," or dominant black. The Dominant Black allele is actually a mutation that
does not allow the agouti gene to be expressed. Because this mutation is dominant, a dog only needs to have one copy of the mutation to suppress the agouti locus. A dog that has one or two copies of the Dominant Black allele will only express his base coat color, which is determined by the B-Locus and E-Locus. He will not express any colors that occur from the agouti gene, such as "black and tan" or "tricolor."The second allele is known as the "brindling" allele, and is represented as "Kbr." The Kbr allele is a separate mutation that still allows the agouti gene to be expressed, however, causes brindling of the agouti patterns. The agouti gene represents several different colors, such as fawn/sable, tricolor, tan points, or recessive black.The Kbr allele is recessive to the KB allele, however, it is dominant over a third allele, Ky. Thus, for a dog to express the brindle pattern, he must be either Kbr/Kbr or Kbr/Ky. Dogs that are KB/Kbr will not appear brindle, but can still pass on that allele and potentially produce brindle offspring.
The third allele is represented as "Ky." This allele allows the agouti gene to be expressed without brindling. When a dog is Ky/Ky at the K-locus, the agouti locus determines the dog's coat color. For example, a dog that is Ay/Ay at the agouti locus could be fawn/sable. If that same dog is KB/KB at the K-locus, the agouti locus will be hidden, and his coloration will be determined at the B- and E- loci. However, if that same dog is Ky/Ky at the K-locus, he will then be able to express agouti, and will be fawn/sable.
At this time, there is no direct test for the "Kbr" allele, although it can generally be inferred through testing for the presence of the Dominant Black allele, as well as through phenotypes of the parents and offspring. Testing for the Dominant Black mutation determines if the dog is able to express agouti phenotypes, however, it is limited in that it will not tell you if the dog will be brindled.
DO YOU REALLY HAVE A BRINDLE |
Description |
Animal Genetics currently offers a test for the K-Locus to determine how many copies of the dominant "KB" allele a dog carries.
Cost
$40.00 US for the KB-allele test.
Sample Collection
Collect sample using buccal swabs provided by Animal Genetics. Ensure that the dog has not eaten within a few hours of sample collection. Any food particles can inhibit the test. Rub each of the swabs along the inside of the dog's mouth for 10-15 seconds, and allow the swabs to dry thoroughly. Label the provided envelope with the dog's name, and place the swab inside it. Download and complete a submission form for each sample and send along with payment to Animal Genetics for testing.
Results
Results are given using the following symbolic notation:
KB-Allele Results:
KB/KB | The dog carries two copies of the dominant "KB" allele. The dog will be not be brindled or express his agouti phenotype. The dog will always pass on a copy of the "KB" allele to all offspring. |
KB/n | One copy of the dominant black allele was detected. The dog will be dominant black, and will not express his agouti phenotype. The dog could pass on this allele, or either the brindle or fawn allele, to any offspring. |
n/n | The dog does not carry the dominant black mutation. The dog's coat color will be determined by the agouti gene, and may be brindled or not brindled. |
Want to know the difference between a RED Pomeranian and an Orange Pomeranian? Canine Color Testing- E Locus | |||||||||||||||||||||||||
Description MC1R, also known as the extension gene, controls production of pigment in melanocytes. The dominant form of the gene, the "E" allele, allows the dog to produce eumelanin, which is a black pigment. A mutation in the MC1R gene causes the pigment-producing cells to only produce phaeomelanin, which is a yellow pigment. This form of the gene is represented as the "e" allele. The "e" allele is recessive, meaning that a dog must have two copies of the MC1R mutation to express the solid yellow coat color. A third allele exists in the extension gene, "EM" which is also dominant. This causes the dog to have a black mask on their face, also known as a melanistic mask. This allele acts similarly to the "E" allele, in that it causes a black-based coat. Because it is dominant, a dog only needs one copy of the "EM" allele to express this trait. In solid black dogs with a copy of the "EM" allele, the mask is hidden, however, it can still pass on the melanistic mask to future offspring. The "ee" genotype can vary in expression between different breeds. In some breeds, the difference between a black or brown dog and a yellow dog is obvious, such as in Labrador Retrievers. However, in other breeds, such as Cocker Spaniels, this difference may be more subtle. Other breeds such as pomeranians express the "ee" phenotype as a red color. It is important to note that the Extension gene is only one of 4 important genes in determining the coat color of a canine. The dogs color can vary greatly with different alleles at other gene. Dogs that are "ee" will always be yellow (base), however, there is a great deal of variation of dogs that are "EE" or "Ee", depending on the B-Locus, A-Locus, and K-Locus.
Cost
E-Allele Results:
EM-Allele Results:
Combination Test Results:
|
Melanin, Agouti, and Red
This is quite a complex topic but its understanding is fundamental to understanding the basic color genetics of the dog. Many of the other genes are simple recessives that have easy-to-understand effects.
Melanin is the substance or pigment that gives a dog's hair its color.There are two distinct types of melanin in the dog - as in many mammals - eumelanin and phaeomelanin. Eumelanin is, in the absence of other modifying genes (see below), black or dark brown. Phaeomelanin is, in its unmodified form, a yellowish color.
Melanin is produced by cells called melanocytes. These are found in the skin, hair bulbs (from which the hairs grow) and other places.
Melanocytes in the skin are sensitive to UV radiation and normally produce eumelanin. They are responsible e.g. for tanning in humans, and for darker exposed skin e.g. nose leather in furred mammals.
Melanocytes within the hair follicles cause melanin to be added to the hair as it grows. However, melanin is not added at a constant 'rate'. At the very tip of the hair, (eu)melanin production is usually most intense, resulting in the darker tip thats frequently seen.
A protein called the Agouti protein has a major effect on the 'injection' of melanin into the growing hair. This is widespread in many mammals, though not in humans. The Agouti protein causes a banding effect on the hair: it causes a fairly sudden change from the production of eumelanin (black/brown pigment) to phaeomelanin (red/yellow pigment). The result is the agouti appearance as typified by the wild rabbit. The term 'Agouti' actually refers to a South American rodent that exemplifies this type of hair.
NOTE: It is worth mentioning at this point that there remain areas of considerable doubt about the exact genes/alleles that affect certain aspects of coat color in dogs. The main ones surround the proposed 'dominant black' (As) at the top of the Agouti series, and in the E series there are questionmarks over Em (black mask) and Ebr (brindle).
In the past it was thought that with the A and E series, you had:
Agouti Series (A): As, ay, aw, as, at, a
Extension Series (E): Em, E, Ebr, e
But newer research indicates that 'dominant black' As may not belong in the Agouti series at all - and this would be consistent with other mammals that also share the Agouti series, none of which has a dominant phaeomelanin suppressor like As at the top of the series. Research also indicates that brindle (Ebr in the older system) and 'dominant black' form a separate series of at least 3 alleles, which can be called the K series (for blacK); this includes both dominant black and brindle.
We have chosen to adopt this later model.
The Agouti Locus - A
The Agouti locus controls the formation of the Agouti protein, that in turn is one of the mechanisms that controls the replacement of eumelanin with phaeomelanin in the growing hair. The alleles of the Agouti locus can affect not just whetheror not the eumelanin->phaeomelanin shift occurs, but also where on the dog's body this happens.
The probable alleles at the Agouti locus, in order of decreasing dominance, are: Ay, aw, as, at and a.
Dominant Black. Dogs certainly do have a dominant form of black that is indeed very dominant: completely obliterating all formation of phaeomelanin pigment. Traditionally, dominant black has been placed at the head of the Agouti series (symbolAs). It is now believed to be part of a separate series (the K series - see below) and not at the Agouti locus at all. This is in keeping with the operation of the Agouti locus in all other mammals that have it: increasing dominance of Agouti locus alleles results in increasing production of phaeomelanin without exception. We mention it here simply because it has long been thought, mistakenly, to be part of the Agouti locus.
So at the top of the Agouti series then we have Ay, Sable - also known as 'dominant yellow' or 'golden sable'. This results in an essentially phaeomelanic phenotype, but the hair tips are eumelanin (black). The extent of the eumelanin tip varies considerably from lighter sables (where just the ear tips are black) to darker sables - where much of the body is dark. It is possible that Ay is not completely dominant over the lower Agouti series alleles, with an Ay heterozygote e.g. Ayat having a darker body. AyAy may be called 'clear red' whereas Ayat can be 'sabled red'. Sable is a very common color in many breeds of dog, e.g. German Shepherd.
Next we have aw, 'wolf' color. This is like Ay but the tan is replaced with a pale gray/cream color and the hairs usually have several bands of light and dark color, not just the black tip of sable. Seen in the Keeshond, Siberian and Norwegian Elkhound.
Moving down the series we next come to as, 'saddle tan'. This is somewhat like the black+tan allele (below), except that eumelanin is restricted to the back and side regions, hence the name 'saddle'. It is also possible that this is due to another gene interacting with at/at genotypes.
Allele at, 'black+tan' is next. This is a primarily black dog but with tan (phaeomelanin) markings around the eyes, muzzle, chest, stomach and lower legs. Commonly seen in hounds, Doberman's and Rottweilers.
Finally, at the bottom of the Agouti series is recessive black, symbol a. When a dog is homozygous for recessive black (aa), it will have no phaeomelanin in its coat (unless it is also ee, which is epistatic to the Agouti series - see below). Examples of breeds that exhibit recessive black are German Shepherd and Shetland Sheepdog. Whilst some breeders discount the presence of a recessive black at the bottom of the Agouti series is is consistent with the behaviour of this locus in many other mammals.
The existence of all these alleles in the Agouti series is not certain, nor is the precise order of dominance of the intermediate alleles aw as and at.
The Extension Locus - E
This refers to the extension of eumelanin over the dog's body. The dominant form, E, is known as normal extension. The recessive form, e, is called non-extension. When a dog is homozygous for non-extension (ee), its coat will be entirely phaeomelanin based - i.e. red/yellow. For this reason this is sometimes termed recessive red or recessive yellow, to distinguish it from the sable reds/yellows generated by the Ay allele at the Agouti locus.
The extension locus is shared by many mammals, e.g. horses, and operates in a very similar way to this description. In the past it had been proposed that there were additional alleles present at the E locus for black mask and brindle, but this is not borne out by breeding data.
The (Dominant) Black Locus - K
This relative newcomer to the traditional models of dog color genetics codes for both dominant black and brindle in decreasing order of dominance: K=dominant blacK, kbr=brindle, k='normal'. So a dog that is KK or Kkbr is dominant black,kbrkbr or kbrk is brindled, and kk is 'normal'.
Brindling is the presence of 'stripes' of eumelanin-based hairs in areas that are otherwise phaeomelanin based. When a dog is brindled the color of the eumelanin stripes may be modified by the normal genes that affect eumelanin (B and D, see below).
Dominant black (K) is epistatic to whatever is found at the Agouti locus, however it in turn is overridden by ee at the Elocus.
Bringing it Together
It appears that the three loci E, K and A act together as follows:
- If a dog is ee at the E locus, its coat will be entirely phaeomelanin based (red/yellow);
- Otherwise (it is Ee or EE at the E locus), if it is K- at the K locus its coat will be entirely eumelanin based (dominant black);
- Otherwise (it is Ee or EE at the E locus), if it is kbrkbr or kbrk at the K locus it will be brindled with the color of the phaeomelanin part of the brindling in turn affected by the Agouti alleles present;
- Otherwise (it is Ee or EE at the E locus, and it is kk at the K locus), the distribution of eumelanin and phaeomelanin will be determined solely by the Agouti alleles present.
With this topic under our belt we can now move on the describing the genes one by one.
Other Genes
Chocolate - B gene
This gene has a lightening effect on eumelanin only. I.e, it has no effect on red-based colors.
In the dog there are two alleles for this gene, with symbols B and b respectively. When B is present (BB or Bb) the brown/black eumelanin is its normal, unlightened, color. But when a dog is bb the brown is lightened to Chocolate.
Blue Dilution - D gene
This recessive gene has a diluting effect on both eumelanin and phaeomelanin. When present in the homozygous recessive form (dd) it dilutes brown eumelanin to blue, and red to cream.
Combinations of B and D in Eumelanistic Coats
The effects of these 2 genes combine to form a range of 4 eumelanistic ('black-based') colors:
Choc | Dilute | Eumelanistic Color |
B- | D- | Black |
B- | dd | Blue |
bb | D- | Liver/Chocolate |
bb | dd | Faded Liver/Chocolate |
Albino - C gene
This gene affects the intensity of melanin production in the coat hairs. The normal or dominant form, C, is what might be termed 'full color'. There are however various incompletely dominant mutant alleles postulated for this locus, with varying effects on color intensity. These mutant forms are temperature sensitive - the higher the temperature, the more effective they are (i.e, the lighter the color).
Almost all dogs are CC at this locus - full color.
The lower series alleles that have been suggested include, in order of decreasing dominance, cch, ce, cb and c.
The first, cch, is chinchilla. This lightens most or all of the phaemelanin with little or no effect on eumelanin. E.g. it turns black+tan to black+silver.
The next allele, ce, is 'extreme dilution'. Causes tan to become almost white. It is thought that the white labrador might bece with another, lower, C series allele. The ce allele may be responsible for producing white hair in other breeds of dogs, like the West Highland White Terrier, while allowing full expression of dark nose and eye pigment
Moving on down we have cb, or blue-eyed albino. This is an entirely white coat but with a very small amount of residual pigment in the eyes, giving pale blue eyes. This is known as ca in cats. Can be called platinum or silver.
Finally we have c, true pink-eyed albino. This doesn't seem to occur in dogs.
Graying - G gene
This is a dominant mutant gene that causes the dog to gray with age - pigmented hairs are progressively replaced with unpigmented hairs.
Super-Extension - Se
This dominant gene controls the expression of a black mask.
Traditional models of dog color genetics placed black mask in the Extension (E) series. However it seems that a more accurate model is to place it at a separate locus. Alternate literature has used the symbol Ma for this.
Most breeds do not exhibit black mask, and are therefore sese for this locus. Breeds that have black mask (Se-) include the Mastiff, Pug and Belgian sheepdog.
White Spotting - S
The piebalding or 'white spotting' gene is common to many mammals e.g. cats, not just dogs. And, as in cats, it is not really fully understood.
In dogs it is thought there are four alleles, in decreasing order of dominance: S, si, sp and sw. The S series alleles appear to be incompletely dominant, further complicating matters. E.g. Ssw will be similar to sisi in appearance. The degree of white spotting is also affected by modifiers. E.g when merle (M) is also present, the white spotting has a greater effect (more white).
The most dominant allele, S, means 'solid color'. Most dogs that are homozygous for S (i.e. SS) have no white hair at all, or possible a tiny amount e.g. a white tail tip, though this can be considered a fault in breeds that are normally SS.
The next allele, si, mean 'irish spotting'. This involves white spotting on most parts of the coat, but not crossing the back.
Moving on down we next come to sp, 'piebald'. The white is more extensive than irish spotting, and often crosses the back.
The most recessive allele in the series is sw, or 'extreme white piebald'. A dog that is homozygous for sw will be almost entirely white, e.g. the Dalmatian.
Breeds that exhibit white spotting use varying terminology for the differing degrees of white. E.g. in herding dogs the terms 'normal white pattern' (sisi), 'white-factored' (sisw) and 'color headed' (swsw) are seen. In Boxers there is the term 'flashy white' (Ssw - si is not thought to exist in the Boxer). The term 'Mantle' is applied to a Great Dane that is sisw.
Dogs exhibiting extensive white spotting are more likely to suffer from deafness than non-white dogs, e.g. Dalmatians.
Ticked - T
This dominant mutation causes the presence of color in those areas that have been made white by the effect of alleles in the white spotting (S) series. An extreme example of ticking is the Dalmatian.
Merle - M
This is an incomplete dominant that causes 'merling' - patchy dilution e.g. black becoming patched with gray ('blue merled') or sable becoming sable merle. Merling has little or no effect on phaeomelanin. The M allele is not found in all breeds; in fact most don't have it. Examples of breeds that display merling include the Australian Shepherd and Dachshund (where it is called 'dapple'). Merling also affects the eye color.
Most merled dogs are actually the heterozygote, Mm. There is good reason for this; there are serious health problems associated with the homoygote MM, including tiny eyes (microopthalmic) or even entirely missing eyes (anopthamlic). The homozygote is also known as a 'double merle'. They are often predominantly white, hence their alternate name 'defective white'.
When breeding merles, many reputable breeders avoid breeding Mm to Mm; they generally go for Mm X mm and get 50% single merles (Mm) and 50% not.
GENOTYPES AND COLORS: ("-" is either the dominant or recessive allele)
AGOUTI:
NON-EXTENSION RED (cream):
|
HORSE COLOR GENETICS
Homozygous creams ("double dilutes")
When a horse is homozygous, meaning it has two copies of the cream allele, the strongest color dilution occurs.
- Cremellos are homozygous cream chestnuts, and have a cream colored body with a cream or white mane and tail.
- Perlinos are homozygous cream bays, which also have a cream-colored body but a mane and tail that may be somewhat more reddish in color than a cremello.
- Smoky Creams are homozygous cream blacks, and very difficult to visually distinguish from cremellos or perlinos.
All three shades can be difficult to distinguish from one another, and are oftentimes only firmly identified after a DNA test. While both red and black pigments are turned cream, the black pigment retains a little more color and tends to have a reddish or rusty tint.[7] Thus all-red coats are turned all-ivory, all-black coats are turned all-rusty cream, and bay coats have ivory bodies with slightly darker points.[3]
Horses with two copies of the cream allele can be collectively called double-dilutes, homozygous creams, or blue-eyed creams, and they share a number of characteristics. The eyes are pale blue, paler than the unpigmented blue eyes associated with white color or white markings, and the skin is rosy-pink. The true, unpigmented pink skin associated with white markings is clearly visible against the rosy-pink skin of a double-dilute, especially when their coat is wetted down. The palest shades of double-dilute coats are just off-white, while the darkest are distinctly rust-tinged. Their coats may be described as nearly white[1] or ivory[3] in addition to cream.
The off-white coat, pale blue eyes, and rosy pink skin distinguish the coats of double-dilutes from those of true white horses. True white horses have unpigmented skin and hair due to the incomplete migration ofmelanocytes from the neural crest during development.[citation needed]
No health defects are associated with the cream gene. This is also true of the normal variations in skin, hair and eye color encoded on the human MATP gene.[8]True white coat coloring can be produced by at least half a dozen known genes, and some are associated with health defects. Some genes which encode a white or near-white coat when heterozygous, popularly called "dominant white," may be lethal in homozygote embryos.[9] Another specific mutation on the endothelin receptor type B (EDNRB) gene is associated with theframe overo pattern produces Lethal white syndrome if homozygous, but carriers can be identified with a DNA test.